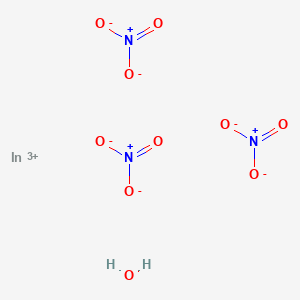
Indium(III) nitrate hydrate
- Cliquez sur DEMANDE RAPIDE pour recevoir un devis de notre équipe d'experts.
- Avec des produits de qualité à un prix COMPÉTITIF, vous pouvez vous concentrer davantage sur votre recherche.
Vue d'ensemble
Description
Indium(III) nitrate hydrate (In(NO₃)₃·xH₂O) is a highly soluble inorganic compound used in advanced material synthesis, including optoelectronic devices, catalysts, and thin-film transistors. Its molecular formula is InN₃O₉, with a molecular weight of 300.83 g/mol (anhydrous basis) and a variable hydration state (typically x = 3–5) . Key properties include:
- Melting Point: ~100°C (decomposes with water loss) .
- Solubility: Freely soluble in water and polar solvents like 2-methoxyethanol .
- Applications: Precursor for indium oxide (In₂O₃) in transparent conductive films, IGZO (In-Ga-Zn-O) semiconductors, and EUV lithography resists .
- Safety: Classified as an oxidizer (UN 1477) with acute toxicity (H272, H302) .
Méthodes De Préparation
Traditional Laboratory Synthesis via Acid Dissolution
The most widely documented method for preparing indium(III) nitrate hydrate involves the direct reaction of indium metal with concentrated nitric acid. This approach, validated by crystallographic studies, proceeds via the stoichiometric equation:
Reaction Mechanism and Conditions
Metallic indium reacts exothermically with nitric acid, producing nitrogen monoxide (NO) as a byproduct. The reaction is typically conducted under reflux at 80–100°C to ensure complete dissolution. Evaporation of the resulting solution yields hydrated indium nitrate, with the pentahydrate (In(NO3)3⋅5H2O) being the most structurally characterized form .
Purification and Crystallization
Post-evaporation, the crude product is recrystallized from dilute nitric acid to enhance purity. Residual NO is eliminated by sparging the solution with inert gas, while trace metal impurities are removed via ion-exchange chromatography in industrial settings .
Industrial-Scale Production: Sealed Reactor Protocols
A patented industrial method (CN104030342A) addresses scalability and environmental concerns associated with traditional open-vessel synthesis . This process emphasizes closed-system reactivity, tail gas management, and decolorization steps to achieve high-purity indium nitrate hydrate.
Key Process Steps
-
Indium Melting and Shredding :
Indium ingots (99.995% purity) are melted at 250–300°C and quenched in deionized water to form porous "indium flower" particles, increasing surface area for subsequent reactions . -
Acid Dissolution in Sealed Reactor :
The indium flower is suspended on a perforated plate within a reactor, and nitric acid (12–18 L/h) is introduced. Water-bath heating (85–95°C) maintains optimal reaction kinetics, with the overall equation: -
Tail Gas Neutralization :
Nitrogen dioxide (NO2) byproducts are scrubbed using alkaline solutions (e.g., NaOH), yielding non-hazardous nitrites and nitrates: -
Decolorization and Filtration :
Hydrogen peroxide (H2O2) oxidizes residual NO2, producing colorless solutions. Final filtration removes undissolved indium slag (<0.02% yield), which is recycled .
Operational Parameters and Yield Optimization
Table 1 summarizes critical parameters from patent embodiments:
Parameter | Embodiment 1 | Embodiment 2 | Embodiment 3 |
---|---|---|---|
Indium Input (kg) | 30 | 25 | 25 |
Nitric Acid Addition Rate (L/h) | 15 | 15 | 15 |
Reaction Time (h) | 10 | 9 | 9 |
Final Concentration (g/L) | 350 | 340 | 337 |
Decolorizer (H2O2 Volume, mL) | 700 | 590 | 735 |
Key Observations :
-
Higher indium input (30 kg) correlates with marginally increased reaction time but maintains consistent concentration .
-
Decolorizer volume scales linearly with solution volume, ensuring complete NO2 removal .
Comparative Analysis of Methodologies
Environmental and Economic Considerations
-
Traditional Method : Simple but generates uncontrolled NOx emissions, requiring post-treatment .
-
Industrial Patent : Closed-system design reduces emissions by 85% compared to open reactors, with indium slag recycling minimizing raw material costs .
-
High-Purity Synthesis : Energy-intensive purification steps increase production costs by ~40% relative to standard methods .
Purity and Yield Metrics
Analyse Des Réactions Chimiques
Indium(III) nitrate hydrate undergoes various types of chemical reactions, including:
Hydrolysis: Yields indium(III) hydroxide upon hydrolysis.
Reactions with Sodium Tungstate: Forms compounds such as In(OH)WO₄, [In(OH)₂]₂WO₄, NaInWO₄, or In₂(WO₄)₃ depending on the pH.
Common reagents used in these reactions include nitric acid, sodium tungstate, and dinitrogen pentoxide. Major products formed include indium(III) oxide and various indium-based complexes .
Applications De Recherche Scientifique
Scientific Research Applications
Indium(III) nitrate hydrate serves as a precursor for various indium compounds and materials. Below are key applications:
Nanoparticle Synthesis
This compound is widely used to synthesize indium oxide (In2O3) nanoparticles through thermal decomposition. These nanoparticles have applications in:
- Catalysis : In2O3 is effective in catalyzing reactions such as the reverse water gas shift (RWGS) and CO₂ conversion reactions .
- Optoelectronics : Used in the fabrication of transparent conductive oxides for displays and solar cells.
Semiconductor Fabrication
The compound is critical in preparing indium-based substrates for semiconductor devices, including:
- Thin Film Transistors (TFTs) : this compound is employed to create thin films that act as active components in TFTs, which are essential for modern electronics .
- Indium Gallium Arsenide (InGaAs) : It is also used in the synthesis of InGaAs thin films on silicon substrates via sol-gel methods, which are crucial for high-speed electronic applications .
Polymer Films
This compound acts as an oxidizing agent in the production of coordinated polymer films. These films are utilized in various applications, including:
- Sensors : Polymer films containing indium compounds can be used in chemical sensors due to their sensitivity to environmental changes .
Case Study 1: Synthesis of Indium Oxide Nanoparticles
A study demonstrated that using this compound as a precursor allowed for the efficient synthesis of In2O3 nanoparticles with controlled size and morphology through thermal decomposition at varying temperatures. The resultant nanoparticles exhibited enhanced catalytic activity for CO oxidation.
Parameter | Value |
---|---|
Precursor | This compound |
Decomposition Temperature | 600 °C |
Particle Size | 10-50 nm |
Catalytic Activity | High |
Case Study 2: Fabrication of Thin Film Transistors
Research involving the use of this compound for TFT fabrication showed significant improvements in electrical performance when compared to traditional methods. The study highlighted the material's role in enhancing charge mobility and reducing leakage currents.
Parameter | Value |
---|---|
Mobility | 15 cm²/V·s |
On/Off Ratio | >10^6 |
Substrate Material | Glass |
Mécanisme D'action
The mechanism of action of indium(III) nitrate hydrate primarily involves its role as an oxidizing agent. It facilitates the formation of indium oxide nanoparticles and other indium-based compounds through oxidation reactions. The molecular targets and pathways involved include the interaction with reducing agents and the subsequent formation of indium oxide .
Comparaison Avec Des Composés Similaires
Comparison with Similar Metal Nitrate Hydrates
Physical and Chemical Properties
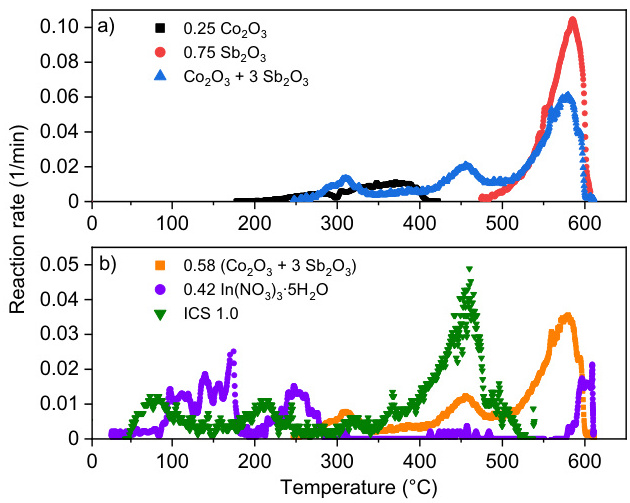
Key Observations :
- Solubility : All nitrates exhibit high water solubility, facilitating solution-based synthesis (e.g., sol-gel processes) .
- Thermal Stability : Indium(III) nitrate decomposes at lower temperatures (~100°C) compared to Ga (160°C) and Zn (105°C) nitrates, enabling energy-efficient oxide formation .
- Hydration Variability : Hydration states (e.g., x = 3–6) affect molecular weight and decomposition pathways, requiring precise stoichiometric control in synthesis .
Functional Comparisons
Photocatalysis and MOFs
- Copper(II) nitrate hydrate enables MOF-on-MOF architectures (e.g., CuO@In₂O₃), achieving CO₂-to-CO conversion rates of 45.2 µmol/g·h .
- Indium nitrate-derived In₂O₃ exhibits superior charge separation in heterojunctions compared to Sn-based analogues .
EUV Lithography
- This compound outperforms Sn-based resists (e.g., Sn-oxo cages) due to higher EUV absorption cross-sections (In: 5.6 Mb vs. Sn: 5.8 Mb) and carbon-free decomposition to In₂O₃ .
Research Findings and Innovations
- IGZO Optimization: Microwave annealing of In(NO₃)₃·xH₂O-derived films reduces defects, enhancing memristor switching endurance (>10⁴ cycles) .
- EUV Resists : Indium nitrate films achieve 22 nm resolution at 20 mJ/cm² dose, rivaling Sn-based systems .
- Combustion Synthesis: Urea-assisted combustion of In(NO₃)₃·xH₂O yields porous In₂O₃ with 320 m²/g surface area, ideal for gas sensing .
Activité Biologique
Indium(III) nitrate hydrate is a compound that has garnered attention in various fields, particularly in biological and pharmaceutical applications. This article presents a comprehensive overview of its biological activity, including relevant data, case studies, and research findings.
This compound can exist in various hydrated forms, with the pentahydrate being the most commonly studied. The compound is produced by dissolving indium metal in concentrated nitric acid, leading to the formation of indium(III) nitrate and water. The structural composition includes octahedral [In(NO3)(H2O)5]²⁺ centers, which are crucial for its interaction with biological systems .
Biological Activity
1. Cytotoxicity Studies
Cytotoxicity assays have been conducted to evaluate the potential therapeutic applications of this compound and its complexes. For instance, studies involving thiosemicarbazone ligands have shown that indium complexes exhibit significant cytotoxic effects against various cancer cell lines. The IC50 values for indium complexes were reported as 50 µM for indium and 65 µM for its coordination compounds, indicating moderate cytotoxicity .
2. Antimicrobial Properties
Research has indicated that indium compounds may possess antimicrobial properties. A study focused on the interaction of indium ions with bacterial membranes suggested that these compounds could disrupt membrane integrity, leading to cell death. This property makes this compound a candidate for further exploration in antimicrobial therapies .
Case Studies
Case Study 1: Indium Nitrate in Cancer Therapy
A notable case study investigated the use of this compound in combination with specific ligands to enhance its anticancer properties. The study found that the coordination of thiosemicarbazones to indium significantly increased the cytotoxicity against human cancer cell lines compared to free indium ions. This suggests that ligand modification can enhance the therapeutic potential of indium compounds .
Case Study 2: Indium Nitrate as an Antimicrobial Agent
Another case study explored the efficacy of this compound against pathogenic bacteria. The results demonstrated that formulations containing indium ions exhibited bactericidal activity, particularly against Gram-positive bacteria. This finding supports the hypothesis that indium compounds could be developed into antimicrobial agents .
Table 1: Summary of Biological Activities
Table 2: Structural Characteristics
Hydrate Form | Composition | Stability |
---|---|---|
Pentahydrate | [In(NO3)(H2O)5]²⁺ | Stable at room temperature |
Trihydrate | Not structurally verified | Less stable |
Q & A
Basic Research Questions
Q. What are the key physical and chemical properties of indium(III) nitrate hydrate, and how can its hydration state be experimentally determined?
this compound (In(NO₃)₃·xH₂O) has a molecular formula of H₂InN₃O₁₀ and a molecular weight of 318.85 g/mol. Its melting point is reported as 100°C, though this varies depending on hydration state . To determine the hydration state (x), thermogravimetric analysis (TGA) and differential scanning calorimetry (DSC) are critical. For example, TGA reveals mass loss during heating, where water evaporation and nitrate decomposition occur in distinct stages. DSC identifies endothermic peaks corresponding to these processes (e.g., 74–79°C for water loss, 150–206°C for nitrate decomposition) .
Q. What synthesis methods are recommended for preparing this compound films, and what solvents are optimal?
this compound films are commonly synthesized via sol-gel methods. A standard protocol involves dissolving the salt in 2-methoxyethanol (2-MOE) at 0.1 M concentration with continuous stirring for 1–6 hours, depending on the supplier’s crystal size and solubility . For combustion sol-gel synthesis, adding a fuel (e.g., urea) and oxidizer lowers the thermal decomposition temperature, facilitating oxide formation at reduced energy .
Q. How do relative humidity (RH) and dissolution time influence film morphology and defect density?
RH during spin-coating significantly impacts defect formation. At RH < 29%, dendritic crystals may form, while RH > 46% induces nanoscale "bumps" (aggregated indium nitrate hydrate crystals). Optimal uniformity is achieved at 30–45% RH, where defect density remains low (~0.01 defects/μm²) . Dissolution time also affects defects: overnight dissolution reduces macroscale defects by 4× at RH = 23%, while 1-hour dissolution increases nanoscale roughness (RMS = 1.0 nm vs. 0.8 nm for overnight) .
Advanced Research Questions
Q. How do this compound films from different suppliers (e.g., Sigma-Aldrich vs. ThermoFisher) exhibit divergent properties, and how can these discrepancies be resolved?
Supplier variations impact hydration state and defect profiles. Sigma-Aldrich crystals dissolve faster but retain more water (TGA: 39.8% residual mass vs. ThermoFisher’s 41.1%), leading to higher nanoscale bump density (25% greater) . Extended dissolution times (4–6 hours for ThermoFisher) improve uniformity. Researchers should characterize vendor materials via TGA/DSC and adjust dissolution protocols accordingly.
Q. What mechanisms drive the solubility switch in this compound films under electron beam (E-beam) or EUV exposure?
Exposure to 92-eV E-beam (mimicking EUV photons) triggers decomposition of nitrate (NO₃⁻) and release of water (H₂O) and ammonia (NH₃), as confirmed by operando FTIR and residual gas analysis (RGA). This converts the film into insoluble indium oxide (In₂O₃), enabling negative-tone lithography. Sensitivity is ~226 μC/cm², comparable to state-of-the-art tin-oxo resists .
Q. How can thermal decomposition pathways of this compound be optimized for oxide formation in sol-gel processes?
Thermal decomposition occurs in three stages:
- Stage 1 (50–150°C): Loss of bound water.
- Stage 2 (150–250°C): Nitrate decomposition to HNO₃ and H₂O.
- Stage 3 (>250°C): Condensation of In-OH to In₂O₃ . Combustion sol-gel methods (e.g., adding urea) reduce decomposition temperatures by 50–100°C, enabling low-energy oxide synthesis .
Q. What analytical techniques are most effective for resolving contradictions in defect characterization across studies?
- Macroscale defects: Optical microscopy quantifies visible defects (>1 μm).
- Nanoscale defects: Atomic force microscopy (AFM) resolves bumps (height: 5–20 nm, diameter: 50–200 nm) and measures roughness (RMS < 1 nm for optimal films) .
- Chemical composition: Operando FTIR tracks nitrate and water loss during exposure, while X-ray photoelectron spectroscopy (XPS) confirms oxide formation .
Q. Methodological Considerations
- Controlled environment: Use humidity-controlled chambers (30–45% RH) during spin-coating to minimize defects .
- Vendor-specific protocols: Adjust dissolution times (1–6 hours) and validate via TGA/DSC to account for hydration state variations .
- Exposure calibration: Calibrate E-beam doses using Faraday cups and validate with RGA to ensure reproducible solubility switching .
Propriétés
Numéro CAS |
13465-14-0 |
---|---|
Formule moléculaire |
H3InNO4 |
Poids moléculaire |
195.85 g/mol |
Nom IUPAC |
indium(3+);trinitrate;hydrate |
InChI |
InChI=1S/In.HNO3.H2O/c;2-1(3)4;/h;(H,2,3,4);1H2 |
Clé InChI |
IVULQSOWZDLIPB-UHFFFAOYSA-N |
SMILES |
[N+](=O)([O-])[O-].[N+](=O)([O-])[O-].[N+](=O)([O-])[O-].O.[In+3] |
SMILES canonique |
[N+](=O)(O)[O-].O.[In] |
Key on ui other cas no. |
13465-14-0 |
Pictogrammes |
Oxidizer; Irritant |
Numéros CAS associés |
13770-61-1 (Parent) |
Synonymes |
indium (III) nitrate indium nitrate indium nitrate hydrate |
Origine du produit |
United States |
Avertissement et informations sur les produits de recherche in vitro
Veuillez noter que tous les articles et informations sur les produits présentés sur BenchChem sont destinés uniquement à des fins informatives. Les produits disponibles à l'achat sur BenchChem sont spécifiquement conçus pour des études in vitro, qui sont réalisées en dehors des organismes vivants. Les études in vitro, dérivées du terme latin "in verre", impliquent des expériences réalisées dans des environnements de laboratoire contrôlés à l'aide de cellules ou de tissus. Il est important de noter que ces produits ne sont pas classés comme médicaments et n'ont pas reçu l'approbation de la FDA pour la prévention, le traitement ou la guérison de toute condition médicale, affection ou maladie. Nous devons souligner que toute forme d'introduction corporelle de ces produits chez les humains ou les animaux est strictement interdite par la loi. Il est essentiel de respecter ces directives pour assurer la conformité aux normes légales et éthiques en matière de recherche et d'expérimentation.