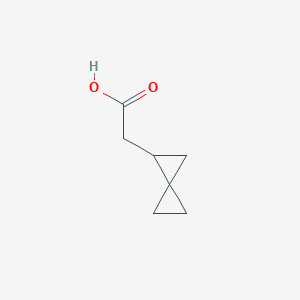
Spiropentaneacetic acid
Vue d'ensemble
Description
Spiropentaneacetic acid is a unique organic compound characterized by a spiropentane ring attached to an acetic acid moiety. This compound is known for its specific inhibitory effects on medium-chain acyl-CoA dehydrogenase, making it a valuable tool in biochemical research.
Applications De Recherche Scientifique
Spiropentaneacetic acid has several applications in scientific research:
Chemistry: Used as a specific inhibitor in studies involving medium-chain acyl-CoA dehydrogenase.
Biology: Helps in understanding metabolic pathways involving fatty acid oxidation.
Medicine: Potential therapeutic applications in conditions related to fatty acid metabolism.
Industry: Used in the synthesis of specialized chemicals and as a research tool in biochemical studies.
Mécanisme D'action
Méthodes De Préparation
Synthetic Routes and Reaction Conditions: The synthesis of spiropentaneacetic acid typically involves the formation of the spiropentane ring followed by the introduction of the acetic acid group. One common method includes the cyclization of a suitable precursor to form the spiropentane ring, followed by carboxylation to introduce the acetic acid functionality. The reaction conditions often involve the use of strong bases and controlled temperatures to ensure the stability of the spiropentane ring.
Industrial Production Methods: Industrial production of this compound may involve similar synthetic routes but on a larger scale. The process would require optimization of reaction conditions to maximize yield and purity. This could include the use of continuous flow reactors and advanced purification techniques such as chromatography.
Analyse Des Réactions Chimiques
Types of Reactions: Spiropentaneacetic acid undergoes various chemical reactions, including:
Oxidation: The acetic acid moiety can be oxidized to form corresponding carboxylates.
Reduction: The compound can be reduced to form alcohol derivatives.
Substitution: The hydrogen atoms on the spiropentane ring can be substituted with other functional groups under appropriate conditions.
Common Reagents and Conditions:
Oxidation: Common oxidizing agents include potassium permanganate and chromium trioxide.
Reduction: Reducing agents such as lithium aluminum hydride or sodium borohydride are typically used.
Substitution: Halogenation reagents like bromine or chlorine can be used for substitution reactions.
Major Products:
Oxidation: Carboxylates and other oxidized derivatives.
Reduction: Alcohol derivatives.
Substitution: Halogenated this compound derivatives.
Comparaison Avec Des Composés Similaires
Methylenecyclopropaneacetic acid: Inhibits both medium-chain and short-chain acyl-CoA dehydrogenase.
Hexyl-substituted methylenecyclopropaneacetic acid: Shows different inhibition specificities for various acyl-CoA dehydrogenases.
Uniqueness: Spiropentaneacetic acid is unique in its specific inhibition of medium-chain acyl-CoA dehydrogenase without affecting amino acid metabolism. This specificity makes it a valuable tool for studying fatty acid oxidation pathways.
Propriétés
IUPAC Name |
2-spiro[2.2]pentan-2-ylacetic acid | |
---|---|---|
Source | PubChem | |
URL | https://pubchem.ncbi.nlm.nih.gov | |
Description | Data deposited in or computed by PubChem | |
InChI |
InChI=1S/C7H10O2/c8-6(9)3-5-4-7(5)1-2-7/h5H,1-4H2,(H,8,9) | |
Source | PubChem | |
URL | https://pubchem.ncbi.nlm.nih.gov | |
Description | Data deposited in or computed by PubChem | |
InChI Key |
QCHONAIUFANQKE-UHFFFAOYSA-N | |
Source | PubChem | |
URL | https://pubchem.ncbi.nlm.nih.gov | |
Description | Data deposited in or computed by PubChem | |
Canonical SMILES |
C1CC12CC2CC(=O)O | |
Source | PubChem | |
URL | https://pubchem.ncbi.nlm.nih.gov | |
Description | Data deposited in or computed by PubChem | |
Molecular Formula |
C7H10O2 | |
Source | PubChem | |
URL | https://pubchem.ncbi.nlm.nih.gov | |
Description | Data deposited in or computed by PubChem | |
DSSTOX Substance ID |
DTXSID90931975 | |
Record name | (Spiro[2.2]pentan-1-yl)acetic acid | |
Source | EPA DSSTox | |
URL | https://comptox.epa.gov/dashboard/DTXSID90931975 | |
Description | DSSTox provides a high quality public chemistry resource for supporting improved predictive toxicology. | |
Molecular Weight |
126.15 g/mol | |
Source | PubChem | |
URL | https://pubchem.ncbi.nlm.nih.gov | |
Description | Data deposited in or computed by PubChem | |
CAS No. |
14364-43-3 | |
Record name | Spiropentaneacetic acid | |
Source | ChemIDplus | |
URL | https://pubchem.ncbi.nlm.nih.gov/substance/?source=chemidplus&sourceid=0014364433 | |
Description | ChemIDplus is a free, web search system that provides access to the structure and nomenclature authority files used for the identification of chemical substances cited in National Library of Medicine (NLM) databases, including the TOXNET system. | |
Record name | (Spiro[2.2]pentan-1-yl)acetic acid | |
Source | EPA DSSTox | |
URL | https://comptox.epa.gov/dashboard/DTXSID90931975 | |
Description | DSSTox provides a high quality public chemistry resource for supporting improved predictive toxicology. | |
Retrosynthesis Analysis
AI-Powered Synthesis Planning: Our tool employs the Template_relevance Pistachio, Template_relevance Bkms_metabolic, Template_relevance Pistachio_ringbreaker, Template_relevance Reaxys, Template_relevance Reaxys_biocatalysis model, leveraging a vast database of chemical reactions to predict feasible synthetic routes.
One-Step Synthesis Focus: Specifically designed for one-step synthesis, it provides concise and direct routes for your target compounds, streamlining the synthesis process.
Accurate Predictions: Utilizing the extensive PISTACHIO, BKMS_METABOLIC, PISTACHIO_RINGBREAKER, REAXYS, REAXYS_BIOCATALYSIS database, our tool offers high-accuracy predictions, reflecting the latest in chemical research and data.
Strategy Settings
Precursor scoring | Relevance Heuristic |
---|---|
Min. plausibility | 0.01 |
Model | Template_relevance |
Template Set | Pistachio/Bkms_metabolic/Pistachio_ringbreaker/Reaxys/Reaxys_biocatalysis |
Top-N result to add to graph | 6 |
Feasible Synthetic Routes
A: Spiropentaneacetic acid acts as a specific inhibitor of medium-chain acyl-CoA dehydrogenase (MCAD) []. MCAD is an enzyme crucial for the breakdown of medium-chain fatty acids, a key energy source for the body. By inhibiting MCAD, SPA disrupts the flow of fatty acid oxidation, leading to the accumulation of dicarboxylic acids, which are then excreted in the urine (dicarboxylic aciduria) []. This effect is observed both in vitro, using rat liver mitochondria, and in vivo, in live rats []. Interestingly, unlike the related compound methylenecyclopropaneacetic acid (MCPA), SPA's inhibitory action appears specific to fatty acid metabolism, with no observed impact on amino acid pathways [].
A: While the provided abstracts don't delve into detailed spectroscopic characterization, they do offer insights into the structure-activity relationship. Notably, SPA's structure, unlike MCPA, doesn't lend itself to forming a ring-opened nucleophile []. MCPA is proposed to exert irreversible inhibition by forming a covalent bond with the FAD prosthetic group of acyl-CoA dehydrogenases []. In contrast, SPA is thought to form a tight, but non-covalent, complex with MCAD, leading to its inhibitory effects []. This difference in binding mode likely contributes to the observed specificity of SPA towards MCAD compared to the broader inhibitory profile of MCPA.
A: Research suggests that the inhibition of fatty acid oxidation by SPA is partially reversible []. Administration of either glycine or L-carnitine, both involved in fatty acid metabolism, has been shown to partially counteract SPA's inhibitory action []. The exact mechanism of this reversal remains to be fully elucidated.
Avertissement et informations sur les produits de recherche in vitro
Veuillez noter que tous les articles et informations sur les produits présentés sur BenchChem sont destinés uniquement à des fins informatives. Les produits disponibles à l'achat sur BenchChem sont spécifiquement conçus pour des études in vitro, qui sont réalisées en dehors des organismes vivants. Les études in vitro, dérivées du terme latin "in verre", impliquent des expériences réalisées dans des environnements de laboratoire contrôlés à l'aide de cellules ou de tissus. Il est important de noter que ces produits ne sont pas classés comme médicaments et n'ont pas reçu l'approbation de la FDA pour la prévention, le traitement ou la guérison de toute condition médicale, affection ou maladie. Nous devons souligner que toute forme d'introduction corporelle de ces produits chez les humains ou les animaux est strictement interdite par la loi. Il est essentiel de respecter ces directives pour assurer la conformité aux normes légales et éthiques en matière de recherche et d'expérimentation.