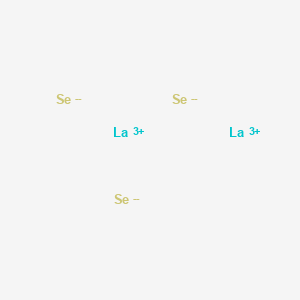
Séléniure de lanthane
Vue d'ensemble
Description
Lanthanum selenide is a chemical compound composed of lanthanum and selenium. It is known for its unique properties and potential applications in various fields, including materials science and electronics. Lanthanum selenide is typically represented by the formula LaSe, indicating a one-to-one ratio of lanthanum to selenium atoms.
Applications De Recherche Scientifique
Lanthanum selenide has several scientific research applications due to its unique properties:
-
Materials Science: Lanthanum selenide is used in the development of advanced materials, including semiconductors and superconductors. Its unique electronic properties make it a valuable component in these materials.
-
Electronics: Lanthanum selenide is used in the fabrication of electronic devices, including transistors and sensors
-
Catalysis: Lanthanum selenide is used as a catalyst in various chemical reactions, including hydrogenation and oxidation reactions. Its high catalytic activity and stability make it a valuable catalyst in these processes.
-
Biomedical Applications: Lanthanum selenide is being explored for its potential use in biomedical applications, including drug delivery and imaging
Mécanisme D'action
Target of Action
Lanthanum selenide is a compound that has been studied for its potential applications in various fields, including electronics and optics . It’s important to note that the targets can vary depending on the specific application or context in which lanthanum selenide is used.
Mode of Action
The mode of action of lanthanum selenide is largely dependent on its physical and chemical properties. For instance, in the context of thin film technology, lanthanum substrates have been shown to improve the crystallinity of copper selenide films by increasing the crystallite size and decreasing both the microstrains and defect density . This suggests that lanthanum selenide may interact with its targets (in this case, the copper selenide films) by influencing their structural properties.
Biochemical Pathways
Selenium-containing molecules are known to play crucial roles in diverse organisms . Two known routes for selenium incorporation into proteins and nucleic acids are via selenocysteine and 2-selenouridine
Pharmacokinetics
Studies on related compounds, such as lanthanum carbonate, indicate that lanthanum has low oral bioavailability and is excreted predominantly in bile
Result of Action
The results of lanthanum selenide’s action can vary depending on the specific context. For instance, in the field of thin film technology, the use of lanthanum substrates has been shown to redshift the energy band gap and double the dielectric constant values of copper selenide films . This suggests that lanthanum selenide may have significant effects on the optical and electrical properties of materials.
Action Environment
The action of lanthanum selenide can be influenced by various environmental factors. For instance, high-temperature stability of different compositions of lanthanum selenide is strongly dependent on pressure: as pressure decreases and temperature increases, the selenium content is reduced and the composition tends to La3Se4 . This suggests that environmental conditions such as temperature and pressure can significantly influence the action, efficacy, and stability of lanthanum selenide.
Méthodes De Préparation
Synthetic Routes and Reaction Conditions: Lanthanum selenide can be synthesized through several methods, including solid-state reactions and chemical vapor deposition. One common method involves the reaction of lanthanum metal with selenium at high temperatures. The reaction is typically carried out in an inert atmosphere to prevent oxidation: [ \text{2 La (s) + 3 Se (s) → 2 LaSe (s)} ]
Industrial Production Methods: In industrial settings, lanthanum selenide is often produced using high-temperature solid-state reactions. The reactants, lanthanum metal and selenium, are mixed in stoichiometric amounts and heated in a sealed container to prevent contamination. The reaction is usually conducted at temperatures ranging from 800°C to 1000°C.
Analyse Des Réactions Chimiques
Types of Reactions: Lanthanum selenide undergoes various chemical reactions, including oxidation, reduction, and substitution reactions.
-
Oxidation: Lanthanum selenide can be oxidized to form lanthanum oxide and selenium dioxide: [ \text{2 LaSe (s) + 3 O}_2 \text{(g) → 2 La}_2 \text{O}_3 \text{(s) + 2 SeO}_2 \text{(g)} ]
-
Reduction: Lanthanum selenide can be reduced by hydrogen gas to form lanthanum metal and hydrogen selenide: [ \text{LaSe (s) + H}_2 \text{(g) → La (s) + H}_2 \text{Se (g)} ]
-
Substitution: Lanthanum selenide can undergo substitution reactions with halogens to form lanthanum halides and selenium: [ \text{LaSe (s) + Cl}_2 \text{(g) → LaCl}_3 \text{(s) + Se (s)} ]
Common Reagents and Conditions: Common reagents used in these reactions include oxygen, hydrogen, and halogens. The reactions are typically carried out at elevated temperatures to ensure complete conversion of the reactants.
Major Products Formed: The major products formed from these reactions include lanthanum oxide, selenium dioxide, lanthanum metal, hydrogen selenide, lanthanum halides, and elemental selenium.
Comparaison Avec Des Composés Similaires
Lanthanum selenide can be compared with other similar compounds, such as lanthanum sulfide and lanthanum telluride.
-
Lanthanum Sulfide (LaS): Lanthanum sulfide has similar electronic properties to lanthanum selenide but is less stable at high temperatures. It is used in similar applications, including electronics and catalysis.
-
Lanthanum Telluride (LaTe): Lanthanum telluride has higher electrical conductivity than lanthanum selenide but is more reactive. It is used in applications requiring high electrical conductivity, such as superconductors.
Lanthanum selenide is unique in its combination of stability, electrical conductivity, and catalytic activity, making it a valuable compound in various scientific and industrial applications.
Activité Biologique
Lanthanum selenide (LaSe) is a binary compound formed from lanthanum and selenium, primarily recognized for its physical properties, particularly in the fields of superconductivity and materials science. Despite its notable applications in these areas, research into its biological activity remains limited. This article explores the current understanding of lanthanum selenide's biological interactions, synthesizing findings from various studies.
Lanthanum selenide typically crystallizes in a face-centered cubic structure, akin to that of sodium chloride, where each lanthanum cation is surrounded by six selenium anions. This arrangement contributes to its unique electrical and magnetic properties, making it a subject of interest in advanced material applications such as superconductivity .
Biological Activity Overview
Research indicates that lanthanum selenide does not exhibit significant biological activity or function. Its relevance primarily lies in its physical characteristics rather than any direct biological interactions. Current investigations have focused on its electrical and superconductive properties rather than its potential effects on biological systems.
Toxicological Considerations
While lanthanum selenide itself is not known for specific biological activities, it is essential to consider the broader context of rare earth elements (REEs), including lanthanides like lanthanum. Chronic exposure to REEs has been associated with various health concerns, including accumulation in vital organs such as the liver and brain, which can lead to alterations in organ function and histopathology . Although direct toxicity from lanthanum selenide has not been extensively documented, understanding the behavior of lanthanides in biological systems is crucial for assessing safety.
Case Studies and Research Findings
- Synthesis and Characterization : Recent studies have focused on the synthesis of lanthanum-based compounds, including lanthanum selenide. For instance, the co-precipitation method has been utilized to create nanostructures that incorporate lanthanum with other materials like cobalt selenide. These studies often report enhanced catalytic properties and antibacterial potential due to the incorporation of lanthanum .
- Antibacterial Properties : A study investigating lanthanum-doped cobalt selenide nanostructures found significant bactericidal behavior against Staphylococcus aureus. The results indicated that the incorporation of lanthanum improved the surface area and active sites for catalytic degradation, suggesting a potential indirect role of lanthanum compounds in antimicrobial applications .
- Superconductivity Research : Research into the superconducting properties of lanthanum selenide crystals has shown that variations in electron concentration can significantly affect their superconducting behavior. This characteristic is crucial for applications in quantum computing and other advanced technologies .
Data Table: Summary of Biological Activity Research
Propriétés
IUPAC Name |
lanthanum(3+);selenium(2-) | |
---|---|---|
Source | PubChem | |
URL | https://pubchem.ncbi.nlm.nih.gov | |
Description | Data deposited in or computed by PubChem | |
InChI |
InChI=1S/2La.3Se/q2*+3;3*-2 | |
Source | PubChem | |
URL | https://pubchem.ncbi.nlm.nih.gov | |
Description | Data deposited in or computed by PubChem | |
InChI Key |
OPSQCCUNFWHTPJ-UHFFFAOYSA-N | |
Source | PubChem | |
URL | https://pubchem.ncbi.nlm.nih.gov | |
Description | Data deposited in or computed by PubChem | |
Canonical SMILES |
[Se-2].[Se-2].[Se-2].[La+3].[La+3] | |
Source | PubChem | |
URL | https://pubchem.ncbi.nlm.nih.gov | |
Description | Data deposited in or computed by PubChem | |
Molecular Formula |
La2Se3 | |
Source | PubChem | |
URL | https://pubchem.ncbi.nlm.nih.gov | |
Description | Data deposited in or computed by PubChem | |
DSSTOX Substance ID |
DTXSID00923321 | |
Record name | Lanthanum selenide (2/3) | |
Source | EPA DSSTox | |
URL | https://comptox.epa.gov/dashboard/DTXSID00923321 | |
Description | DSSTox provides a high quality public chemistry resource for supporting improved predictive toxicology. | |
Molecular Weight |
514.7 g/mol | |
Source | PubChem | |
URL | https://pubchem.ncbi.nlm.nih.gov | |
Description | Data deposited in or computed by PubChem | |
CAS No. |
12031-51-5 | |
Record name | Lanthanum selenide (La2Se3) | |
Source | ChemIDplus | |
URL | https://pubchem.ncbi.nlm.nih.gov/substance/?source=chemidplus&sourceid=0012031515 | |
Description | ChemIDplus is a free, web search system that provides access to the structure and nomenclature authority files used for the identification of chemical substances cited in National Library of Medicine (NLM) databases, including the TOXNET system. | |
Record name | Lanthanum selenide (La2Se3) | |
Source | EPA Chemicals under the TSCA | |
URL | https://www.epa.gov/chemicals-under-tsca | |
Description | EPA Chemicals under the Toxic Substances Control Act (TSCA) collection contains information on chemicals and their regulations under TSCA, including non-confidential content from the TSCA Chemical Substance Inventory and Chemical Data Reporting. | |
Record name | Lanthanum selenide (2/3) | |
Source | EPA DSSTox | |
URL | https://comptox.epa.gov/dashboard/DTXSID00923321 | |
Description | DSSTox provides a high quality public chemistry resource for supporting improved predictive toxicology. | |
Record name | Dilanthanum triselenide | |
Source | European Chemicals Agency (ECHA) | |
URL | https://echa.europa.eu/substance-information/-/substanceinfo/100.031.581 | |
Description | The European Chemicals Agency (ECHA) is an agency of the European Union which is the driving force among regulatory authorities in implementing the EU's groundbreaking chemicals legislation for the benefit of human health and the environment as well as for innovation and competitiveness. | |
Explanation | Use of the information, documents and data from the ECHA website is subject to the terms and conditions of this Legal Notice, and subject to other binding limitations provided for under applicable law, the information, documents and data made available on the ECHA website may be reproduced, distributed and/or used, totally or in part, for non-commercial purposes provided that ECHA is acknowledged as the source: "Source: European Chemicals Agency, http://echa.europa.eu/". Such acknowledgement must be included in each copy of the material. ECHA permits and encourages organisations and individuals to create links to the ECHA website under the following cumulative conditions: Links can only be made to webpages that provide a link to the Legal Notice page. | |
Avertissement et informations sur les produits de recherche in vitro
Veuillez noter que tous les articles et informations sur les produits présentés sur BenchChem sont destinés uniquement à des fins informatives. Les produits disponibles à l'achat sur BenchChem sont spécifiquement conçus pour des études in vitro, qui sont réalisées en dehors des organismes vivants. Les études in vitro, dérivées du terme latin "in verre", impliquent des expériences réalisées dans des environnements de laboratoire contrôlés à l'aide de cellules ou de tissus. Il est important de noter que ces produits ne sont pas classés comme médicaments et n'ont pas reçu l'approbation de la FDA pour la prévention, le traitement ou la guérison de toute condition médicale, affection ou maladie. Nous devons souligner que toute forme d'introduction corporelle de ces produits chez les humains ou les animaux est strictement interdite par la loi. Il est essentiel de respecter ces directives pour assurer la conformité aux normes légales et éthiques en matière de recherche et d'expérimentation.