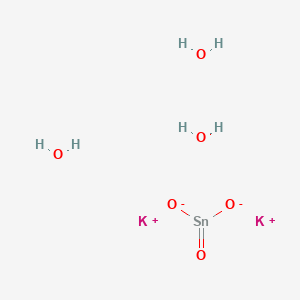
Stannate de potassium trihydraté
Vue d'ensemble
Description
Potassium stannate trihydrate, with the chemical formula K₂SnO₃·3H₂O, is a tin-based inorganic compound. It is commonly used in various industrial applications, particularly in electroplating and as a catalyst. This compound is known for its high purity and stability, making it a valuable reagent in both laboratory and industrial settings .
Applications De Recherche Scientifique
Potassium stannate trihydrate has a wide range of scientific research applications:
Chemistry: It is used as a catalyst in organic synthesis and as a reagent in analytical chemistry.
Biology: It is employed in the preparation of biological samples for electron microscopy.
Medicine: It is investigated for its potential use in drug delivery systems and as an antimicrobial agent.
Industry: It is widely used in electroplating, particularly for tin and its alloys, improving surface brightness, adhesion, and corrosion resistance. .
Mécanisme D'action
Target of Action
Potassium stannate trihydrate is primarily recognized for its utility in industrial processes and materials science research . As a source of tin(IV) ions, it is frequently used in electroplating to deposit tin onto various substrates . This enhances solderability and corrosion resistance .
Mode of Action
It is known that the compound provides a source of tin(iv) ions, which interact with the substrate during the electroplating process . The tin ions are reduced at the substrate surface, forming a layer of tin metal that enhances the substrate’s properties .
Biochemical Pathways
In the context of electroplating, the compound plays a role in the electrochemical reactions that occur at the substrate surface .
Pharmacokinetics
As an industrial chemical, it is typically handled with protective equipment to minimize exposure .
Result of Action
The primary result of potassium stannate trihydrate’s action in electroplating is the formation of a tin layer on the substrate . This layer enhances the substrate’s solderability and corrosion resistance .
Action Environment
The action of potassium stannate trihydrate is influenced by various environmental factors. For instance, the efficiency of the electroplating process can be affected by the pH, temperature, and concentration of the electroplating solution . Additionally, the compound’s stability and efficacy can be influenced by storage conditions .
Analyse Biochimique
Biochemical Properties
It is known that as a source of tin (IV) ions, it can interact with various biomolecules in the context of industrial processes
Cellular Effects
It is primarily used in industrial settings rather than biological contexts
Molecular Mechanism
As a source of tin (IV) ions, it may interact with biomolecules at the molecular level . The specifics of these interactions, including any binding interactions with biomolecules, enzyme inhibition or activation, and changes in gene expression, are not currently known.
Temporal Effects in Laboratory Settings
In laboratory settings, Potassium stannate trihydrate has been found to possess a large CO2 uptake capacity The long-term effects of this compound on cellular function in in vitro or in vivo studies have not been reported
Méthodes De Préparation
Synthetic Routes and Reaction Conditions: Potassium stannate trihydrate can be synthesized through several methods. One common approach involves the reaction of metallic tin with concentrated nitric acid to form beta-stannic acid. This intermediate is then reacted with potassium hydroxide to produce potassium stannate. The reaction conditions typically involve heating and controlled addition of reagents to ensure complete conversion .
Industrial Production Methods: In industrial settings, potassium stannate trihydrate is often produced using tin stripping waste liquid. This method involves converting crude tin hydroxide to high-purity tin hydroxide, which is then reacted with potassium hydroxide under controlled conditions to yield potassium stannate trihydrate. This process is environmentally friendly and cost-effective, producing high-purity crystals suitable for various applications .
Analyse Des Réactions Chimiques
Types of Reactions: Potassium stannate trihydrate undergoes several types of chemical reactions, including:
Oxidation: It can be oxidized to form higher oxidation state tin compounds.
Reduction: It can be reduced to lower oxidation state tin compounds.
Substitution: It can participate in substitution reactions where the tin atom is replaced by other metal atoms.
Common Reagents and Conditions:
Oxidation: Common oxidizing agents include hydrogen peroxide and potassium permanganate.
Reduction: Reducing agents such as sodium borohydride and hydrazine are often used.
Substitution: Reactions typically involve metal salts and are conducted in aqueous or organic solvents.
Major Products:
Oxidation: Tin dioxide (SnO₂) is a common product.
Reduction: Tin(II) compounds such as tin(II) chloride.
Substitution: Various metal stannates depending on the substituting metal.
Comparaison Avec Des Composés Similaires
- Sodium stannate trihydrate (Na₂SnO₃·3H₂O)
- Calcium stannate (CaSnO₃)
- Lithium stannate (Li₂SnO₃)
Comparison: Potassium stannate trihydrate is unique due to its high solubility in water and its ability to produce high-quality coatings in electroplating applications. Compared to sodium stannate trihydrate, it offers better conductivity and surface finish. Calcium stannate and lithium stannate, while useful in other applications, do not provide the same level of performance in electroplating and catalytic processes .
Potassium stannate trihydrate’s versatility and effectiveness in various applications make it a valuable compound in both research and industry.
Activité Biologique
Potassium stannate trihydrate (K₂SnO₃·3H₂O) is an inorganic compound that has garnered attention for its potential applications in various fields, particularly in environmental chemistry and materials science. This article delves into the biological activity of potassium stannate trihydrate, focusing on its properties, synthesis methods, and its role in carbon dioxide capture, as well as its potential implications for human health and the environment.
Potassium stannate trihydrate is a white crystalline solid that is soluble in water. It is synthesized through a series of chemical reactions involving tin compounds and alkali. A common method involves the neutralization of tin tetrachloride with potassium hydroxide, resulting in high-purity potassium stannate with minimal toxic byproducts . The compound has a molecular weight of approximately 298.94 g/mol and decomposes at temperatures above 140 °C .
Carbon Dioxide Capture
Recent studies have highlighted the effectiveness of potassium stannate as a sorbent for carbon dioxide (CO₂) capture. Laboratory tests have demonstrated that solid-state synthesized potassium stannate exhibits superior CO₂ uptake capacities compared to other stannates. For instance, commercial K₂SnO₃ showed a maximum CO₂ uptake of 2.77 mmol CO₂/g (12.2 wt%) at 700 °C, while in-house synthesized variants achieved even higher efficiencies .
The kinetics of CO₂ adsorption on potassium stannate were characterized by a rapid initial uptake, with one variant (K-B) displaying an adsorption rate significantly higher than its counterparts—0.016 mg/s after just five minutes of exposure to CO₂ . This rapid adsorption is attributed to the compound's structural properties and the effective diffusion of potassium ions during the sorption process.
Table 1: Summary of CO₂ Sorption Capacities of Potassium Stannate Variants
Sample | Maximum CO₂ Uptake (wt%) | Adsorption Rate (mg/s) | Temperature (°C) |
---|---|---|---|
K-B | 7.3 | 0.016 | 800 |
Commercial K₂SnO₃ | 12.2 | 0.11 | 700 |
K-C | 6.3 | N/A | 850 |
K-D | 6.1 | N/A | 800 |
Study on High-Temperature Performance
In a recent study, various forms of potassium stannate were tested under different thermal conditions to assess their CO₂ capture capabilities. The results indicated that increasing temperature enhances the sorption performance due to improved diffusion rates within the material . Notably, K-B maintained consistent performance across multiple cycles, indicating its potential for sustainable applications in carbon capture technologies.
Health and Safety Considerations
While potassium stannate trihydrate is primarily recognized for its industrial applications, its biological activity warrants consideration regarding human exposure and environmental impact. The compound's low toxicity profile suggests it may be safer than other heavy metal compounds; however, comprehensive toxicological studies are necessary to fully understand its implications for human health and ecosystems .
Propriétés
IUPAC Name |
dipotassium;dioxido(oxo)tin;trihydrate | |
---|---|---|
Source | PubChem | |
URL | https://pubchem.ncbi.nlm.nih.gov | |
Description | Data deposited in or computed by PubChem | |
InChI |
InChI=1S/2K.3H2O.3O.Sn/h;;3*1H2;;;;/q2*+1;;;;;2*-1; | |
Source | PubChem | |
URL | https://pubchem.ncbi.nlm.nih.gov | |
Description | Data deposited in or computed by PubChem | |
InChI Key |
HTHDWDSBYOUAFF-UHFFFAOYSA-N | |
Source | PubChem | |
URL | https://pubchem.ncbi.nlm.nih.gov | |
Description | Data deposited in or computed by PubChem | |
Canonical SMILES |
O.O.O.[O-][Sn](=O)[O-].[K+].[K+] | |
Source | PubChem | |
URL | https://pubchem.ncbi.nlm.nih.gov | |
Description | Data deposited in or computed by PubChem | |
Molecular Formula |
H6K2O6Sn | |
Source | PubChem | |
URL | https://pubchem.ncbi.nlm.nih.gov | |
Description | Data deposited in or computed by PubChem | |
Molecular Weight |
298.95 g/mol | |
Source | PubChem | |
URL | https://pubchem.ncbi.nlm.nih.gov | |
Description | Data deposited in or computed by PubChem | |
CAS No. |
12125-03-0 | |
Record name | Potassium stannate trihydrate | |
Source | ChemIDplus | |
URL | https://pubchem.ncbi.nlm.nih.gov/substance/?source=chemidplus&sourceid=0012125030 | |
Description | ChemIDplus is a free, web search system that provides access to the structure and nomenclature authority files used for the identification of chemical substances cited in National Library of Medicine (NLM) databases, including the TOXNET system. | |
Record name | Dipotassium tin trioxide trihydrate | |
Source | European Chemicals Agency (ECHA) | |
URL | https://echa.europa.eu/information-on-chemicals | |
Description | The European Chemicals Agency (ECHA) is an agency of the European Union which is the driving force among regulatory authorities in implementing the EU's groundbreaking chemicals legislation for the benefit of human health and the environment as well as for innovation and competitiveness. | |
Explanation | Use of the information, documents and data from the ECHA website is subject to the terms and conditions of this Legal Notice, and subject to other binding limitations provided for under applicable law, the information, documents and data made available on the ECHA website may be reproduced, distributed and/or used, totally or in part, for non-commercial purposes provided that ECHA is acknowledged as the source: "Source: European Chemicals Agency, http://echa.europa.eu/". Such acknowledgement must be included in each copy of the material. ECHA permits and encourages organisations and individuals to create links to the ECHA website under the following cumulative conditions: Links can only be made to webpages that provide a link to the Legal Notice page. | |
Record name | POTASSIUM STANNATE TRIHYDRATE | |
Source | FDA Global Substance Registration System (GSRS) | |
URL | https://gsrs.ncats.nih.gov/ginas/app/beta/substances/R38Y86O4SY | |
Description | The FDA Global Substance Registration System (GSRS) enables the efficient and accurate exchange of information on what substances are in regulated products. Instead of relying on names, which vary across regulatory domains, countries, and regions, the GSRS knowledge base makes it possible for substances to be defined by standardized, scientific descriptions. | |
Explanation | Unless otherwise noted, the contents of the FDA website (www.fda.gov), both text and graphics, are not copyrighted. They are in the public domain and may be republished, reprinted and otherwise used freely by anyone without the need to obtain permission from FDA. Credit to the U.S. Food and Drug Administration as the source is appreciated but not required. | |
Avertissement et informations sur les produits de recherche in vitro
Veuillez noter que tous les articles et informations sur les produits présentés sur BenchChem sont destinés uniquement à des fins informatives. Les produits disponibles à l'achat sur BenchChem sont spécifiquement conçus pour des études in vitro, qui sont réalisées en dehors des organismes vivants. Les études in vitro, dérivées du terme latin "in verre", impliquent des expériences réalisées dans des environnements de laboratoire contrôlés à l'aide de cellules ou de tissus. Il est important de noter que ces produits ne sont pas classés comme médicaments et n'ont pas reçu l'approbation de la FDA pour la prévention, le traitement ou la guérison de toute condition médicale, affection ou maladie. Nous devons souligner que toute forme d'introduction corporelle de ces produits chez les humains ou les animaux est strictement interdite par la loi. Il est essentiel de respecter ces directives pour assurer la conformité aux normes légales et éthiques en matière de recherche et d'expérimentation.