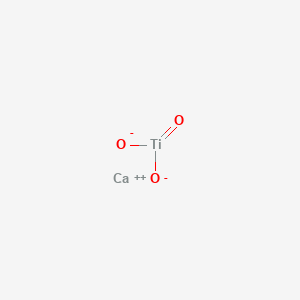
Calcium titanate
Vue d'ensemble
Description
Calcium titanate (CaTiO₃) is a perovskite-type oxide with the general formula ABO₃, where Ca²⁺ occupies the A-site and Ti⁴⁺ the B-site. It crystallizes in three primary phases: orthorhombic (most stable below 1380 K), cubic (1380–1500 K), and tetragonal (above 1500 K) . Key properties include:
- Dielectric Constant: Moderate (~150–170), suitable for capacitors and sensors .
- Band Gap: ~3.5 eV, limiting visible-light photocatalysis but modifiable via doping .
- Thermal Stability: Retains structural integrity up to 1370°C, ideal for high-temperature applications .
- Biocompatibility: Non-toxic and osteogenic, enhancing bone-implant adhesion .
Applications span electronics (capacitors, sensors), photocatalysis (antibiotic degradation), and biomedical engineering (bone repair coatings) .
Méthodes De Préparation
Combustion Synthesis Using Bio-Waste Precursors
Combustion synthesis has emerged as a sustainable approach for calcium titanate production, particularly when utilizing bio-waste materials. A 2019 study demonstrated the synthesis of CaTiO₃ using waste duck eggshells as a calcium source, combined with anatase titanium dioxide (A-TiO₂) and magnesium (Mg) as a reducing agent . The process involved two milling techniques: high-energy planetary milling and conventional ball milling.
High-Energy Milling and Reaction Dynamics
High-energy milling of eggshell and A-TiO₂ for 30 minutes generated a reactive surface area of 12.5 m²/g, compared to 8.2 m²/g for ball-milled precursors . This increased surface area facilitated a complete combustion reaction, yielding a single-phase perovskite structure. Post-synthesis leaching removed residual MgO, and sintering at 1350°C for 180 minutes produced ceramics with a density of 3.65 g/cm³ and porosity of 0.54% .
Dielectric Performance
The sintered CaTiO₃ exhibited a dielectric constant (εᵣ) of 78 and a loss tangent (tan δ) of 0.02 at 1 MHz, attributed to reduced interfacial polarization defects . These properties make combustion-synthesized CaTiO₃ suitable for high-frequency capacitors and resonators.
Table 1: Combustion Synthesis Parameters and Outcomes
Parameter | High-Energy Milling | Ball Milling |
---|---|---|
Surface Area (m²/g) | 12.5 | 8.2 |
Sintering Temperature (°C) | 1350 | 1350 |
Density (g/cm³) | 3.65 | 3.42 |
Porosity (%) | 0.54 | 1.89 |
Sol-Gel Template Method for Morphological Control
The sol-gel method enables precise control over this compound morphology. A 2016 study utilized degreasing cotton as a bio-template to synthesize microtubular CaTiO₃ (CTCTO) . The procedure involved immersing cotton in a sol containing calcium nitrate, tetrabutyl titanate, and polyethylene glycol, followed by calcination at 750–1050°C.
Structural Evolution with Calcination Temperature
-
750°C : Amorphous phase with residual carbon from cotton.
-
900°C : Crystalline perovskite phase (XRD peaks at 2θ = 33.2°, 47.5°) .
-
1050°C : Enhanced crystallinity but partial particle agglomeration.
Functional Applications
CTCTO calcined at 900°C demonstrated superior adsorption capacity for heavy metals (Pb²⁺: 98%, Cd²⁺: 95%) and photocatalytic degradation of methylene blue (89% in 120 minutes) . The microtubular structure provided a high surface area of 68 m²/g, facilitating active site accessibility.
Solid-State Reaction: Conventional vs. Laser Sintering
Conventional Solid-State Synthesis
Traditional solid-state methods involve calcining CaCO₃ and TiO₂ at 1000°C for 8–12 hours, followed by sintering at 1150°C for 10+ hours . This energy-intensive process often results in inhomogeneous grain growth and impurities.
Rapid Laser Sintering
A 2020 study introduced laser sintering of sol-gel-derived precursors to synthesize Zn-doped CaCu₃Ti₄O₁₂ (CCTO) . The process achieved full crystallization in 60 seconds, compared to 20 hours in conventional furnaces. Laser-sintered CCTO exhibited a cubic perovskite structure (a = 7.38 Å) and a dielectric constant of 1.2 × 10⁴ at 1 kHz .
Table 2: Comparison of Sintering Techniques
Parameter | Laser Sintering | Conventional Sintering |
---|---|---|
Time | 60 seconds | 20 hours |
Grain Size (nm) | 85 | 120–150 |
Dielectric Constant (εᵣ) | 12,000 | 10,000 |
Hydrothermal Synthesis for High-Purity Hydrates
A patented hydrothermal method (US5126011A) produces high-purity this compound hydrates (kassite phase) using calcium carbonate and titanium alkoxide . Key steps include:
-
Dispersion of CaCO₃ in ethanol.
-
Coating with titanium alkoxide under vacuum.
-
Hydrothermal treatment at 200°C for 6 hours.
The resulting hexagonal kassite crystals (CaTiO₃·H₂O) exhibited a specific surface area of 45 m²/g and purity >99.5% . This method avoids high-temperature calcination, preserving hydroxyl groups for enhanced photocatalytic activity.
Comparative Analysis of Synthesis Methods
Table 3: Method-Specific Advantages and Limitations
Method | Advantages | Limitations |
---|---|---|
Combustion Synthesis | Low-cost, bio-waste utilization | Requires post-leaching for purity |
Sol-Gel Template | Morphological control | Long calcination times |
Laser Sintering | Rapid processing, energy-efficient | Limited to thin-film applications |
Hydrothermal | High purity, low defect density | High pressure equipment required |
Analyse Des Réactions Chimiques
Decomposition Reactions
At extreme temperatures (>1500°C), CaTiO₃ partially decomposes, releasing oxygen and forming secondary phases :
Doping with Nb₂O₅ accelerates decomposition, forming CaNb₂O₄ above 0.10 mol% dopant concentration :
Substitution Reactions
Ionic substitution modifies CaTiO₃’s electronic and optical properties:
A-Site Substitution (Calcium Replacement)
-
La³⁺ doping : Enhances photocatalytic activity by reducing bandgap energy :
Bandgap decreases from 3.5 eV (pure CaTiO₃) to 2.8 eV (x=0.2) .
B-Site Substitution (Titanium Replacement)
-
Fe³⁺ doping : Improves oxygen reduction reaction (ORR) kinetics in fuel cells :
At x=0.1, ORR activity increases by 40% compared to undoped CaTiO₃ .
Surface Reactions
CaTiO₃’s surface reactivity is critical for biomedical and catalytic applications:
Hydrophilicity and Biocompatibility
-
Hydrothermal treatment : Increases surface hydroxyl groups, improving hydrophilicity (contact angle <30°) .
-
Protein adsorption : Enhanced by surface roughness (Sa >0.8 μm), promoting osteoblast adhesion .
Surface Roughness (Sa, μm) | Contact Angle (°) | Cell Proliferation (vs. Control) | Source |
---|---|---|---|
0.49 | 85 | 100% | |
0.87 | 28 | 145% |
Catalytic Activity
-
Photocatalysis : Doped CaTiO₃ degrades organic pollutants under UV light via hydroxyl radical (·OH) generation :
Titanium Production
CaTiO₃ is reduced to metallic titanium via carbothermal reduction :
Dielectric Composites
Reactions with polymers (e.g., PVDF) enhance dielectric permittivity :
Applications De Recherche Scientifique
Electronics and Dielectrics
High Dielectric Constant Material
Calcium titanate is recognized for its high dielectric constant, which makes it suitable for various electronic applications. It is often used in the manufacturing of capacitors, particularly multilayer ceramic capacitors (MLCCs), where it allows for the miniaturization of electronic components by enabling higher capacitance in smaller volumes. The dielectric constant of this compound can reach values around 1400, depending on processing conditions and material composition .
Table 1: Dielectric Properties of this compound
Property | Value |
---|---|
Dielectric Constant | Up to 1400 |
Quality Factor | 130 - 3000 |
Varistors
The nonlinear dielectric properties of this compound make it an excellent candidate for varistors, which protect electronic circuits from voltage spikes. This application is critical in surge protection devices used in consumer electronics .
Ceramics Industry
This compound is extensively used in the ceramics industry to produce glazes and ceramic materials with enhanced properties.
Ceramic Glazes
In ceramic glazes, this compound contributes to improved durability and aesthetic qualities. The compound allows for precise control over color and texture, which is essential for high-quality ceramic products such as tiles and tableware .
Refractory Materials
this compound enhances the thermal shock resistance of refractory materials used in high-temperature applications like steel production. Its ability to modify porosity and sinterability makes it suitable for creating durable refractories .
Photocatalysis and Environmental Applications
Recent research has highlighted the potential of this compound as a photocatalyst for environmental applications, particularly in the reduction of carbon dioxide. Studies have shown that this compound can effectively facilitate photocatalytic reactions under visible light, contributing to CO2 reduction efforts .
Biomedical Applications
This compound has been investigated for its biocompatibility and potential use in biomedical implants. The material can enhance osteointegration due to its ability to release calcium ions, which are beneficial for bone growth. Research indicates that titanium alloys coated with this compound exhibit improved biological responses compared to uncoated implants .
Nanotechnology
In nanotechnology, this compound nanoparticles are synthesized for various applications, including catalysis and as components in nanocomposites. These nanoparticles exhibit unique electrical and optical properties that are harnessed in advanced materials development .
Case Study 1: High Dielectric Constant Capacitors
A study demonstrated that incorporating this compound into capacitor designs significantly increased capacitance without compromising size. This advancement is crucial for modern electronics where space is limited.
Case Study 2: Photocatalytic Reduction of CO2
Research conducted at Kyoto University explored the use of this compound as a photocatalyst for CO2 reduction. The results showed a marked increase in efficiency when using this compound under visible light conditions, suggesting its potential role in sustainable energy solutions .
Mécanisme D'action
The mechanism by which calcium titanate exerts its effects involves its unique crystalline structure and chemical properties:
Photocatalytic Mechanism: Upon exposure to light, this compound generates electron-hole pairs.
Catalytic Mechanism: As a catalyst support, this compound provides a stable surface for the dispersion of active metal species, enhancing the efficiency of catalytic reactions.
Comparaison Avec Des Composés Similaires
Strontium Titanate (SrTiO₃)
Key Differences :
- SrTiO₃ has a higher dielectric constant but lower thermal stability.
- CaTiO₃ outperforms SrTiO₃ in biomedical applications due to its superior osteogenic properties .
Barium Titanate (BaTiO₃)
Key Differences :
- BaTiO₃ exhibits ferroelectricity and a much higher dielectric constant, making it superior for capacitors.
- CaTiO₃ is preferred in environments requiring chemical resistance and moderate dielectric performance .
Lead Titanate (PbTiO₃)
Property | CaTiO₃ | PbTiO₃ |
---|---|---|
Piezoelectricity | Weak (tunable via doping) | Strong |
Toxicity | Non-toxic | Toxic (Pb²⁺ leaching) |
Applications | Biomedical coatings | Sensors, actuators |
Key Differences :
- PbTiO₃’s toxicity limits biomedical use, whereas CaTiO₃ is safe for bone implants .
- PbTiO₃ has superior piezoelectricity but requires hazardous lead .
Magnesium Titanate (MgTiO₃)
Property | CaTiO₃ | MgTiO₃ |
---|---|---|
Band Gap (eV) | 3.5 | ~3.8 |
Photocatalytic Activity | Moderate (enhanced via doping) | Lower (requires UV light) |
Thermal Stability | High (>1200°C) | Moderate (~1000°C) |
Key Differences :
- MgTiO₃’s larger band gap limits visible-light photocatalysis compared to CaTiO₃ .
- CaTiO₃’s thermal stability favors high-temperature industrial applications .
Calcium Copper Titanate (CaCu₃Ti₄O₁₂, CCTO)
Property | CaTiO₃ | CCTO |
---|---|---|
Dielectric Constant | ~150–170 | ~10,000–100,000 |
Conductivity | Insulating | Semiconducting |
Applications | Sensors, capacitors | High-density energy storage |
Key Differences :
- CCTO’s colossal dielectric constant surpasses CaTiO₃, but it faces challenges in phase purity and stability .
- CaTiO₃ is simpler to synthesize and more cost-effective for moderate-performance devices .
Titanium Dioxide (TiO₂)
Property | CaTiO₃ | TiO₂ |
---|---|---|
Band Gap (eV) | 3.5 | 3.2 (anatase) |
Photocatalytic Efficiency | Lower under visible light | Higher (broader UV activity) |
Biomedical Use | Osteogenic coatings | Limited (no calcium release) |
Key Differences :
Activité Biologique
Calcium titanate (CaTiO₃) is a bioceramic material that has garnered significant attention in biomedical applications, particularly in bone repair and dental implants. Its biological activity is primarily attributed to its biocompatibility, bioactivity, and potential antibacterial properties. This article explores the biological activity of this compound, presenting detailed research findings, data tables, and case studies.
Overview of this compound
This compound is a ceramic compound with a perovskite structure, known for its excellent mechanical properties and biocompatibility. It is used in various medical applications, including bone grafts, dental implants, and drug delivery systems. The material's ability to bond with bone and promote osteogenesis makes it a valuable candidate for orthopedic and dental applications.
Bioactivity and Osteogenic Properties
Bioactivity refers to the ability of a material to elicit a biological response when in contact with living tissue. This compound exhibits bioactive properties by forming a hydroxyapatite layer on its surface when immersed in simulated body fluid (SBF), which is crucial for bone integration.
Case Study: Iodine-Loaded this compound
A study conducted by Ikeda et al. (2022) investigated the bioactivity and antibacterial activity of iodine-containing this compound (Ca-I-Ti). The results showed that:
- Cell Viability : MC3T3-E1 cells seeded on Ca-I-Ti demonstrated high viability and proliferation rates.
- Alkaline Phosphatase (ALP) Activity : The average ALP activity was significantly higher in the Ca-I-Ti group compared to conventional titanium (cp-Ti) groups, indicating enhanced osteogenic potential.
- Antibacterial Activity : Ca-I-Ti exhibited substantial antibacterial effects against methicillin-susceptible Staphylococcus aureus, with a reduction in viable bacterial counts over time .
The study's findings are summarized in the following table:
Parameter | Ca-I-Ti | cp-Ti |
---|---|---|
Cell Viability (%) | 95 ± 2 | 85 ± 3 |
ALP Activity (µg/mL) | 150 ± 10 | 90 ± 5 |
Bacterial Count (CFU × 10⁴) | 0.13 ± 0.18 | 7.3 ± 4.5 |
Antibacterial Properties
The incorporation of iodine into this compound enhances its antibacterial properties. Iodine ions are known for their broad-spectrum antimicrobial activity, which helps prevent infections associated with implants.
Research Findings on Antibacterial Efficacy
In vitro studies have shown that iodine-loaded this compound significantly reduces bacterial colonization compared to traditional titanium surfaces. For instance, after 24 hours of incubation:
- Ca-I-Ti : Bacterial count was reduced to 0.13 CFU × 10⁴.
- cp-Ti : Bacterial count was recorded at 7.3 CFU × 10⁴ .
This significant reduction indicates the potential of iodine-loaded this compound as an effective biomaterial for preventing implant-related infections.
Cytotoxicity and Genotoxicity Studies
Recent studies have also evaluated the cytotoxicity and genotoxicity of this compound nanoparticles (CaTiO₃ NPs). A study examined the effects of CaTiO₃ NPs on mouse hepatic tissue and found that:
- Reactive Oxygen Species (ROS) : Elevated levels were observed in treated groups, indicating oxidative stress.
- Genotoxic Effects : The tail moment in comet assays showed significant DNA damage in treated groups compared to controls .
The following table summarizes the genotoxicity findings:
Treatment | Tail Length (mm) | % DNA in Tail (%) | Tail Moment |
---|---|---|---|
Control | 5.11 ± 0.77 | 33.88 ± 5.99 | 1.90 ± 0.70 |
CaTiO₃ NPs | 4.34 ± 0.45 | 32.84 ± 2.09 | 1.77 ± 0.41 |
Q & A
Q. What are the common synthesis methods for calcium titanate, and how do they influence material properties?
This compound is synthesized via multiple methods, each impacting properties like crystallinity, grain size, and porosity:
- Solid-state reaction : High-temperature calcination of CaCO₃ and TiO₂ yields polycrystalline CaTiO₃, often with larger grain sizes and lower surface area .
- Sol-gel method : Produces nanoparticles with controlled porosity and high purity, ideal for photocatalysis and thin-film applications .
- Hydrothermal synthesis : Enables fine-tuning of morphology (e.g., nanowires or microsheets) by adjusting temperature and pressure .
- Molten salt method : Generates whiskers or polyhedra with enhanced dielectric properties, suitable for electronic ceramics .
Q. Which characterization techniques are critical for analyzing this compound’s structural and morphological properties?
Key techniques include:
- X-ray diffraction (XRD) : Identifies crystallographic phase purity and perovskite structure .
- Scanning electron microscopy (SEM) : Reveals surface morphology (e.g., grain boundaries, porosity) .
- Fourier-transform infrared spectroscopy (FTIR) : Detects functional groups and bonding in sol-gel-derived samples .
- Energy-dispersive X-ray spectroscopy (EDX) : Confirms elemental composition and dopant distribution .
Q. How does doping with elements like Cu or Br influence the dielectric properties of this compound-based ceramics?
Doping modifies crystal structure and electronic behavior:
- Cu/Br co-doping : Reduces loss tangent in CaCu₃Ti₄O₁₂ (CCTO) ceramics while enhancing dielectric constant (>10⁴) via grain boundary engineering .
- Fe doping : Introduces defect states, improving thermal stability for high-temperature capacitors .
Q. What methodologies are employed to assess the biocompatibility of this compound for biomedical implants?
- In vitro cell viability assays : Osteoblast adhesion and proliferation on CaTiO₃ microsheets indicate biocompatibility .
- In vivo studies : Implantation in animal models (e.g., bone grafts) evaluates osteogenesis and inflammatory response .
- Surface modification : Hydroxyapatite coating enhances bioactivity and apatite formation .
Q. How can researchers resolve contradictions in photocatalytic efficiency data across different synthesis routes?
- Control experiments : Standardize testing conditions (light intensity, pollutant concentration) to isolate synthesis impacts .
- Surface area analysis : Compare BET surface area (e.g., sol-gel samples >100 m²/g vs. solid-state <10 m²/g) to explain efficiency variations .
- Band gap measurement : UV-Vis spectroscopy confirms bandgap differences (e.g., 3.5 eV for CaTiO₃ vs. 3.2 eV for TiO₂) .
Q. What safety protocols are essential when handling this compound in laboratory settings?
- Dust control : Use fume hoods and HEPA filters to avoid inhalation .
- Personal protective equipment (PPE) : Nitrile gloves, goggles, and lab coats compliant with NIOSH/EN 166 standards .
- Waste disposal : Collect particles in sealed containers to prevent environmental release .
Q. What mechanisms underlie this compound’s high dielectric constant, and how can they be optimized?
The high dielectric constant arises from:
- Grain boundary effects : Internal barrier layer capacitance in polycrystalline samples .
- Defect engineering : Oxygen vacancies and cation substitutions enhance polarization .
Optimization strategies include doping (e.g., Br/F co-doping) and sintering temperature adjustments to refine grain size .
Q. How do grain size reduction and porosity enhancement improve photocatalytic efficiency?
- Nanostructuring : Smaller grains (<100 nm) increase reactive surface area for pollutant adsorption .
- Mesoporous structures : Facilitate light penetration and charge carrier separation, critical for degrading organic pollutants like amoxicillin .
Q. What role does this compound play in environmental remediation, particularly pollutant degradation?
CaTiO₃ acts as a photocatalyst under UV light:
- Mechanism : Electron-hole pairs generate reactive oxygen species (ROS) that mineralize organic pollutants .
- Modifications : Loading with metals (e.g., Ag) extends activity to visible light .
Q. How do co-doping strategies affect the structural stability and electronic properties of this compound?
Propriétés
IUPAC Name |
calcium;oxygen(2-);titanium(4+) | |
---|---|---|
Details | Computed by LexiChem 2.6.6 (PubChem release 2019.06.18) | |
Source | PubChem | |
URL | https://pubchem.ncbi.nlm.nih.gov | |
Description | Data deposited in or computed by PubChem | |
InChI |
InChI=1S/Ca.3O.Ti/q+2;3*-2;+4 | |
Details | Computed by InChI 1.0.5 (PubChem release 2019.06.18) | |
Source | PubChem | |
URL | https://pubchem.ncbi.nlm.nih.gov | |
Description | Data deposited in or computed by PubChem | |
InChI Key |
WEUCVIBPSSMHJG-UHFFFAOYSA-N | |
Details | Computed by InChI 1.0.5 (PubChem release 2019.06.18) | |
Source | PubChem | |
URL | https://pubchem.ncbi.nlm.nih.gov | |
Description | Data deposited in or computed by PubChem | |
Canonical SMILES |
[O-2].[O-2].[O-2].[Ca+2].[Ti+4] | |
Details | Computed by OEChem 2.1.5 (PubChem release 2019.06.18) | |
Source | PubChem | |
URL | https://pubchem.ncbi.nlm.nih.gov | |
Description | Data deposited in or computed by PubChem | |
Molecular Formula |
CaO3Ti | |
Details | Computed by PubChem 2.1 (PubChem release 2019.06.18) | |
Source | PubChem | |
URL | https://pubchem.ncbi.nlm.nih.gov | |
Description | Data deposited in or computed by PubChem | |
DSSTOX Substance ID |
DTXSID70890839 | |
Record name | Calcium titanium oxide | |
Source | EPA DSSTox | |
URL | https://comptox.epa.gov/dashboard/DTXSID70890839 | |
Description | DSSTox provides a high quality public chemistry resource for supporting improved predictive toxicology. | |
Molecular Weight |
135.94 g/mol | |
Details | Computed by PubChem 2.1 (PubChem release 2021.05.07) | |
Source | PubChem | |
URL | https://pubchem.ncbi.nlm.nih.gov | |
Description | Data deposited in or computed by PubChem | |
CAS No. |
12049-50-2, 37226-56-5 | |
Record name | Calcium titanate | |
Source | ChemIDplus | |
URL | https://pubchem.ncbi.nlm.nih.gov/substance/?source=chemidplus&sourceid=0012049502 | |
Description | ChemIDplus is a free, web search system that provides access to the structure and nomenclature authority files used for the identification of chemical substances cited in National Library of Medicine (NLM) databases, including the TOXNET system. | |
Record name | Calcium titanium oxide | |
Source | EPA DSSTox | |
URL | https://comptox.epa.gov/dashboard/DTXSID70890839 | |
Description | DSSTox provides a high quality public chemistry resource for supporting improved predictive toxicology. | |
Record name | CALCIUM TITANATE | |
Source | FDA Global Substance Registration System (GSRS) | |
URL | https://gsrs.ncats.nih.gov/ginas/app/beta/substances/6RX87EZD0Z | |
Description | The FDA Global Substance Registration System (GSRS) enables the efficient and accurate exchange of information on what substances are in regulated products. Instead of relying on names, which vary across regulatory domains, countries, and regions, the GSRS knowledge base makes it possible for substances to be defined by standardized, scientific descriptions. | |
Explanation | Unless otherwise noted, the contents of the FDA website (www.fda.gov), both text and graphics, are not copyrighted. They are in the public domain and may be republished, reprinted and otherwise used freely by anyone without the need to obtain permission from FDA. Credit to the U.S. Food and Drug Administration as the source is appreciated but not required. | |
Avertissement et informations sur les produits de recherche in vitro
Veuillez noter que tous les articles et informations sur les produits présentés sur BenchChem sont destinés uniquement à des fins informatives. Les produits disponibles à l'achat sur BenchChem sont spécifiquement conçus pour des études in vitro, qui sont réalisées en dehors des organismes vivants. Les études in vitro, dérivées du terme latin "in verre", impliquent des expériences réalisées dans des environnements de laboratoire contrôlés à l'aide de cellules ou de tissus. Il est important de noter que ces produits ne sont pas classés comme médicaments et n'ont pas reçu l'approbation de la FDA pour la prévention, le traitement ou la guérison de toute condition médicale, affection ou maladie. Nous devons souligner que toute forme d'introduction corporelle de ces produits chez les humains ou les animaux est strictement interdite par la loi. Il est essentiel de respecter ces directives pour assurer la conformité aux normes légales et éthiques en matière de recherche et d'expérimentation.