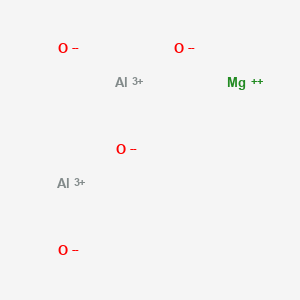
Magnesium aluminate
Vue d'ensemble
Description
Magnesium aluminate (MgAl₂O₄), a spinel-structured ceramic, is the only stable compound in the MgO–Al₂O₃ binary system at ambient pressure . It exhibits exceptional properties, including a high melting point (~2135°C), excellent thermal shock resistance, low thermal expansion coefficient, and resistance to chemical attack, making it indispensable in refractory applications for steel ladles and cement kilns . Its photocatalytic activity (e.g., degrading 88% of methylene blue under UV light in 5 hours) and optical bandgap tunability (2.7–3.8 eV) further position it as a cost-effective alternative to TiO₂ and indium tin oxide (ITO) . Additionally, MgAl₂O₄ serves as an electrolyte in magnesium batteries, where cation aggregation states ([Mg₂Cl₃]⁺, [Mg₃Cl₅]⁺) influence electrochemical performance . Despite its advantages, traditional synthesis methods (e.g., solid-state reactions, sol-gel) often incur high costs, though advanced techniques like laser powder bed fusion (LPBF) are emerging as scalable alternatives .
Méthodes De Préparation
Solid-State Reaction Method
The solid-state reaction route remains the most industrially prevalent method for synthesizing MgAl₂O₄ due to its simplicity and scalability . This technique involves high-temperature calcination of stoichiometric mixtures of magnesium and aluminum precursors, typically oxides or hydroxides.
Reaction Dynamics and Precursor Selection
Key studies have demonstrated that the choice of precursors significantly impacts reaction kinetics and product quality. For instance, Itatani et al. (2006) compared the reactivity of α-Al₂O₃, γ-Al₂O₃, MgO, and Mg(OH)₂ in various combinations . The mixture of γ-Al₂O₃ (31.6 nm primary particles) and Mg(OH)₂ (61.1 nm) exhibited the fastest formation of MgAl₂O₄, achieving near-complete conversion at 1200°C for 1 hour . In contrast, α-Al₂O₃ required higher temperatures (≥1400°C) due to its lower surface area and slower diffusion rates . The decomposition of Mg(OH)₂ to MgO at ~400°C generates reactive amorphous phases, facilitating interparticle diffusion and spinel nucleation .
Phase Evolution and Crystallization
High-temperature X-ray diffraction (HT-XRD) analysis revealed that MgAl₂O₄ formation initiates at 800°C, with crystallinity increasing progressively up to 1200°C . Synchrotron radiation diffraction (SRD) data indicated intermediate phases such as transient γ-Al₂O₃ and MgO during heating, which react to form the spinel structure . The crystallite size of MgAl₂O₄ synthesized via solid-state reactions typically ranges from 50 to 200 nm, depending on calcination duration and precursor morphology .
Table 1: Solid-State Reaction Parameters and Outcomes
Precursors | Calcination Temperature (°C) | Holding Time (h) | Crystallite Size (nm) | Phase Purity (%) |
---|---|---|---|---|
γ-Al₂O₃ + Mg(OH)₂ | 1200 | 1 | 55–75 | 98 |
α-Al₂O₃ + MgO | 1400 | 2 | 120–200 | 95 |
γ-Al₂O₃ + MgO | 1300 | 1.5 | 80–100 | 97 |
Sol-Gel and Hydrothermal-Assisted Synthesis
Sol-gel methods offer superior control over particle size and homogeneity compared to solid-state routes. Tun and Naing (2019) synthesized MgAl₂O₄ nanoparticles via a hydrothermal-assisted sol-gel technique using aluminum nitrate, magnesium chloride, and urea . The process involved gel formation at 160°C for 5 hours, followed by calcination at 800–1150°C .
Crystallization Behavior
XRD analysis confirmed spinel phase formation at 800°C, with crystallite sizes increasing from 10.15 nm (800°C) to 28.02 nm (1150°C) . Fourier-transform infrared (FTIR) spectroscopy identified characteristic Mg–O–Al vibrations at 670 cm⁻¹, affirming spinel structure development . Scanning electron microscopy (SEM) revealed agglomerated nanoparticles with plate-like morphology, attributed to Ostwald ripening during calcination .
Table 2: Hydrothermal Sol-Gel Synthesis Outcomes
Calcination Temperature (°C) | Crystallite Size (nm) | Surface Area (m²/g) | Bandgap Energy (eV) |
---|---|---|---|
800 | 10.15 | 85 | 3.25 |
1000 | 25.77 | 45 | 3.18 |
1150 | 28.02 | 30 | 3.12 |
Gelatin Method: A Low-Cost Alternative
The gelatin method, a bio-inspired synthesis route, employs gelatin as a chelating agent to homogenize metal ions. Figueredo et al. (2017) prepared MgAl₂O₄ using Al(NO₃)₃·9H₂O, Mg(NO₃)₂·6H₂O, and gelatin, followed by calcination at 700–1100°C .
Thermal Decomposition and Crystallinity
Thermogravimetric analysis (TGA) showed a 17.69% weight loss below 600°C, corresponding to gelatin decomposition and nitrate removal . Calcination at 1100°C for 4 hours yielded 90.4% crystallinity with crystallites of 55.5 nm . SEM images displayed a porous microstructure with plate-like aggregates, advantageous for catalytic applications .
Self-Propagating High-Temperature Synthesis (SHS)
SHS leverages exothermic reactions to achieve rapid, energy-efficient synthesis. A recent study utilized Mg–Al alloy (50:50 mass ratio) ignited in air to produce MgAl₂O₄ . The combustion wave propagated at 1500–2000°C, forming phase-pure spinel within minutes . Transmission electron microscopy (TEM) revealed nanocrystallites (20–50 nm) with minimal agglomeration, suitable for optical applications .
Comparative Analysis of Synthesis Methods
Table 3: Method Comparison for MgAl₂O₄ Synthesis
Method | Temperature (°C) | Time | Crystallite Size (nm) | Advantages | Limitations |
---|---|---|---|---|---|
Solid-State Reaction | 1200–1400 | 1–4 h | 50–200 | Low cost, scalable | High energy, large particles |
Hydrothermal Sol-Gel | 160–1150 | 5 h + calc | 10–28 | Homogeneous nanoparticles | Complex steps, high reagent cost |
Gelatin Method | 700–1100 | 2–4 h | 12–55 | Eco-friendly, low-temperature | Low crystallinity at <900°C |
SHS | 1500–2000 | <10 min | 20–50 | Rapid, energy-efficient | Requires precise stoichiometry |
Analyse Des Réactions Chimiques
Solid-State Reaction Between MgO and Al₂O₃
The primary synthesis route involves the reaction of magnesium oxide (MgO) and aluminum oxide (Al₂O₃):
-
Temperature Dependence :
-
Mechanism :
Alternative Methods
-
Co-Precipitation :
-
Self-Propagating High-Temperature Synthesis (SHS) :
Thermal Decomposition and Stability
MgAl₂O₄ decomposes under extreme conditions:
-
Chlorination-Induced Decomposition :
At 1000°C in Cl₂, spinel decomposes into volatile MgCl₂ and Al₂O₃ :
-
High-Pressure Stability :
Under 21–27 GPa , MgAl₂O₄ forms solid solutions with Mg₂SiO₄ (forsterite), stabilizing as calcium ferrite-type structures .
TiO₂ as a Sintering Aid
-
Adding 4 wt.% TiO₂ reduces synthesis temperature to 1400°C and enhances densification .
-
TiO₂ promotes liquid-phase sintering, accelerating Mg²⁺/Al³⁺ diffusion .
Effect of Other Oxides
Comparative Reaction Data
Reaction Kinetics
-
Activation Energy :
-
Crystallite Growth :
Industrial and Functional Reactions
Applications De Recherche Scientifique
Magnesium aluminate spinel has gained attention for its optical properties. It possesses excellent mechanical strength and transparency in the visible to infrared range, making it suitable for:
- Optical Components : Lenses and windows for high-performance optical systems.
- Laser Applications : As a substrate material in laser devices due to its durability and thermal stability .
Biocompatibility in Medical Applications
Recent advancements have explored the use of this compound in biomedical fields. Its biocompatibility makes it a candidate for:
- Implant Materials : Used in biodegradable implants due to its ability to support bone growth while gradually degrading in the body.
- Surface Coatings : Enhancing the corrosion resistance of magnesium alloys through micro-arc oxidation (MAO) treatment, which incorporates this compound coatings to improve mechanical properties and biocompatibility .
Application Area | Properties Utilized | Specific Use Cases |
---|---|---|
Implant Materials | Biocompatibility, biodegradability | Temporary scaffolds for wound healing |
Surface Coatings | Corrosion resistance | Coatings for magnesium alloys |
Synthesis and Processing Techniques
The synthesis of this compound can be achieved through various methods including:
- Molten Salt Method : Produces nanoparticles with controlled size and morphology.
- Solid-State Reaction : Involves high-temperature reactions between magnesium oxide and aluminum oxide.
These methods influence the properties of the final product, such as particle size distribution, crystallinity, and phase purity .
Case Study 1: Development of Refractory Crucibles
A recent study investigated the use of MANPs in manufacturing refractory crucibles. The research demonstrated that crucibles made from MANPs exhibited superior thermal insulation properties with minimal weight loss at elevated temperatures. The study concluded that MANPs are promising materials for developing efficient melting crucibles used in metallurgical processes .
Case Study 2: Optical Applications
Research into the optical properties of MgAl₂O₄ revealed its potential as a substrate material for advanced optical devices. The study highlighted its durability under extreme conditions and its effectiveness in maintaining optical clarity across a range of temperatures .
Mécanisme D'action
The mechanism of action of spinel in various applications is primarily due to its crystal structure and surface properties . In catalysis, the active sites on the spinel surface facilitate chemical reactions. In biological applications, the small size and surface charge of spinel nanoparticles allow them to interact with biological molecules and cells effectively .
Comparaison Avec Des Composés Similaires
Zinc Aluminate (ZnAl₂O₄)
Structural and Defect Similarities :
ZnAl₂O₄ shares the spinel structure with MgAl₂O₄. Both compounds exhibit Schottky defects, though oxygen vacancies dominate diffusion in MgAl₂O₄, while ZnAl₂O₄’s defect dynamics remain understudied . Doping with Y³⁺ or La³⁺ enhances thermal stability in both, reducing surface energy by ~0.3 J/m² in doped MgAl₂O₄ and inhibiting grain growth in Al-rich ZnAl₂O₄ .
Key Differences :
- Thermal Stability : ZnAl₂O₄ has lower melting points and reduced thermal shock resistance compared to MgAl₂O₄, limiting its use in high-temperature refractories.
- Catalytic Applications: ZnAl₂O₄ nanoparticles show promise in catalysis due to surface segregation effects, whereas MgAl₂O₄ excels in photocatalysis and battery electrolytes .
Cobalt Aluminate (CoAl₂O₄)
Structural Modifications :
CoAl₂O₄ adopts a spinel framework where Co²⁺ occupies tetrahedral sites. MgO doping increases cation disorder, enlarging surface area (up to 150 m²/g) and pore volume, enhancing its utility in ceramic pigments and magnetic materials .
Functional Contrasts :
- Optical Properties : CoAl₂O₄’s blue hue derives from Co²⁺ ligand-field transitions, unlike MgAl₂O₄’s UV transparency.
- Magnetic Behavior: CoAl₂O₄ exhibits paramagnetism, whereas MgAl₂O₄ is non-magnetic .
Magnesium Aluminum Oxynitride (MgAlON)
Compositional Flexibility :
MgAlON’s spinel-type structure allows nitrogen incorporation, enabling tunable mechanical properties (e.g., Vickers hardness up to 15 GPa) and transparency for armor applications .
Synthesis Challenges :
MgAlON requires combustion synthesis at >1600°C, contrasting with MgAl₂O₄’s lower-temperature sol-gel or solid-state routes .
Aluminum Magnesium Hydroxide (Hydrotalcite-like Phases)
Rehydration Behavior :
MgAl₂O₄ can transform into hydrotalcite (Mg₆Al₂(OH)₁₆CO₃·4H₂O) under hydrothermal conditions, a property absent in ZnAl₂O₄ or CoAl₂O₄. This phase shows moderate catalytic activity in sugar isomerization but lacks the thermal stability of spinels .
Data Tables
Table 1. Comparative Properties of Spinel-Structured Aluminates
Activité Biologique
Magnesium aluminate (MgAl₂O₄), commonly referred to as spinel, is a compound of significant interest across various fields, including materials science and biomedicine. This article explores its biological activity, focusing on its synthesis, properties, and applications in biomedical contexts.
Synthesis of this compound
The synthesis of this compound can be achieved through various methods, including sol-gel processes, molten-salt synthesis, and traditional solid-state reactions. Each method influences the properties of the resulting nanoparticles:
- Sol-Gel Method : This approach allows for the controlled synthesis of MgAl₂O₄ nanoparticles with specific characteristics. The use of chelating agents like EDTA and oxalic acid significantly affects the morphology and photocatalytic properties of the nanoparticles .
- Molten-Salt Synthesis : This method has been reported to produce nanocrystalline MgAl₂O₄ with enhanced structural integrity. Optimal conditions include a sintering temperature of 850°C .
Properties of this compound
This compound exhibits several notable properties that contribute to its biological activity:
- Biocompatibility : Studies indicate that MgAl₂O₄ has excellent biocompatibility, making it suitable for biomedical applications such as drug delivery systems and implantable devices .
- Adsorption Capacity : MgAl₂O₄ nanoparticles have shown high adsorption capacities for various pollutants, including heavy metals like Cr(VI). This property is attributed to electrostatic interactions and the generation of active free radicals during photocatalytic processes .
1. Toxicity and Biocompatibility
Research has demonstrated that MgAl₂O₄ exhibits low toxicity in vivo. For instance, studies involving animal models have shown that MgAl₂O₄ does not elicit significant inflammatory responses when implanted in tissues . The material's biocompatibility is crucial for its use in medical applications.
2. Drug Delivery Systems
The high surface area and tunable porosity of MgAl₂O₄ nanoparticles make them ideal candidates for drug delivery systems. They can encapsulate therapeutic agents and release them in a controlled manner, enhancing the efficacy of treatments while minimizing side effects.
3. Bone Regeneration
This compound has been investigated for its potential in bone regeneration. Its ability to support osteogenic differentiation of stem cells makes it a promising candidate for bone scaffolds. Studies have shown that MgAl₂O₄ can enhance the proliferation and differentiation of bone marrow stromal cells (BMSCs), promoting new bone formation .
Case Studies
Several case studies highlight the biological activity of this compound:
- Study on Drug Adsorption : A study demonstrated that MgAl₂O₄ could effectively adsorb various pharmaceutical compounds from aqueous solutions, indicating its potential use in wastewater treatment and pharmaceutical recovery .
- In Vivo Biocompatibility Assessment : In an experiment involving rabbit models, MgAl₂O₄ implants showed no adverse effects on surrounding tissues over a 24-week period. The implants integrated well with bone tissue, supporting their use in orthopedic applications .
Research Findings Summary
Property | Findings |
---|---|
Synthesis Methods | Sol-gel and molten-salt methods yield high-quality nanoparticles with controlled properties. |
Biocompatibility | Low toxicity observed in vivo; suitable for medical applications. |
Adsorption Capacity | High capacity for heavy metal adsorption; effective in environmental remediation. |
Bone Regeneration | Promotes osteogenic differentiation; enhances BMSC proliferation. |
Q & A
Basic Research Questions
Q. What are the common synthesis methods for magnesium aluminate spinel, and how do they influence material properties?
this compound spinel is synthesized via methods including sol-gel, co-precipitation, combustion, hydrothermal, and gelatin-based routes. Each method impacts crystallinity, particle size, and morphology:
- Sol-gel synthesis produces nanoparticles with high surface area, ideal for catalytic applications .
- Gelatin method (using nitrates as precursors) yields single-phase materials with crystallite sizes of 12.4–55.5 nm, where higher calcination temperatures (e.g., 1100°C) enhance crystallinity to ~90% .
- Combustion synthesis offers rapid, energy-efficient processing but may require post-synthesis annealing to improve phase purity .
Q. How do calcination parameters affect the structural properties of this compound spinel?
Calcination temperature and duration directly influence crystallite growth and phase purity. For example:
- At 700°C , incomplete spinel formation occurs, resulting in low crystallinity (~39%).
- At 1100°C , prolonged calcination (4 hours) promotes particle coalescence and reduces lattice defects, achieving >90% crystallinity .
- Additives like LiF or TiO₂ can lower sintering temperatures by 200–300°C, enabling single-step densification .
Advanced Research Questions
Q. How can researchers reconcile contradictions between single-step and two-stage sintering processes for dense MgAl₂O₄ ceramics?
- Two-stage sintering (e.g., pre-firing at 1600°C followed by HIP) mitigates ~8% volume expansion during phase formation, ensuring high density (>99% theoretical) .
- Single-step methods using nano-powders or additives (e.g., Y₂O₃) achieve comparable density by suppressing grain boundary migration, as demonstrated in recent studies .
Methodological Insight: Optimize precursor particle size and doping to balance cost and performance .
Q. What role does cation aggregation play in this compound electrolytes for rechargeable batteries?
In Mg-ion batteries, cationic species like [Mg₂Cl₃·6THF]⁺ and [Mg₃(μ₃-Cl)₂(μ₂-Cl)₃]⁺ influence electrolyte stability and Mg deposition efficiency:
- Mononuclear [MgCl]⁺ enhances ion mobility but reduces oxidative stability.
- Trinuclear aggregates improve Coulombic efficiency (e.g., 95% retention over 100 cycles) by stabilizing the electrolyte-electrode interface .
Data Table: Electrochemical Performance of MgAl₂O₄ vs. Graphite
Current Density (A/g) | MgAl₂O₄ Capacitance (F/g) | Graphite Capacitance (F/g) |
---|---|---|
1 | 115.06 | 14.53 |
10 | 40.07 | 30.17 |
Source: |
Q. How can transparency in MgAl₂O₄ ceramics be optimized for optical applications?
Transparent spinel requires:
- High-purity precursors (e.g., >99.99% Al₂O₃/MgO) to minimize light-scattering impurities .
- Hot isostatic pressing (HIP) at 1900°C under 200 MPa argon to eliminate residual porosity .
- Stoichiometric control : Mg-rich compositions (MgO:Al₂O₃ = 1.05:1) reduce secondary phases, enhancing transmittance to >80% in the IR spectrum .
Q. What mechanisms govern cation mobility in MgAl₂O₄ at high temperatures?
At 1000°C, Mg²⁺ ions migrate under electric fields, forming MgO layers at cathodes. Ionic transference numbers reach ~0.5, critical for applications in solid oxide fuel cells (SOFCs) .
Q. How does this compound perform in CO₂ capture applications?
MgAl₂O₄ exhibits moderate CO₂ adsorption (~1.2 mmol/g at 400°C) but can be enhanced via alkali promotion (e.g., K-doped variants achieve ~2.5 mmol/g) .
Q. What experimental designs address challenges in synthesizing MgAl₂O₄ for LNCM cathode calcination?
- Phase compatibility : MgAl₂O₄ resists Li₂O corrosion up to 1000°C, making it suitable for LNCM cathode saggers .
- Thermal shock resistance : Adding 6 wt% spinel powder reduces crack propagation by 40% .
Q. How do characterization techniques validate spinel formation and quality?
- XRD : Confirms cubic phase (Fd-3m space group) and quantifies crystallinity .
- SEM/TEM : Reveals agglomerate morphology and grain boundaries .
- ESI-MS : Identifies cationic species in electrolytes .
Q. Why do contradictory findings arise in spinel synthesis, and how can they be resolved?
Discrepancies stem from precursor reactivity, sintering atmosphere, and doping. For example:
- Nano-powders reduce sintering temperatures but require strict stoichiometric control to avoid Al-rich secondary phases .
- Additives like TiO₂ improve densification but may alter thermal expansion coefficients .
Recommendation: Standardize synthesis protocols and cross-validate with multiple characterization methods .
Propriétés
IUPAC Name |
dialuminum;magnesium;oxygen(2-) | |
---|---|---|
Details | Computed by Lexichem TK 2.7.0 (PubChem release 2021.05.07) | |
Source | PubChem | |
URL | https://pubchem.ncbi.nlm.nih.gov | |
Description | Data deposited in or computed by PubChem | |
InChI |
InChI=1S/2Al.Mg.4O/q2*+3;+2;4*-2 | |
Details | Computed by InChI 1.0.6 (PubChem release 2021.05.07) | |
Source | PubChem | |
URL | https://pubchem.ncbi.nlm.nih.gov | |
Description | Data deposited in or computed by PubChem | |
InChI Key |
UAMZXLIURMNTHD-UHFFFAOYSA-N | |
Details | Computed by InChI 1.0.6 (PubChem release 2021.05.07) | |
Source | PubChem | |
URL | https://pubchem.ncbi.nlm.nih.gov | |
Description | Data deposited in or computed by PubChem | |
Canonical SMILES |
[O-2].[O-2].[O-2].[O-2].[Mg+2].[Al+3].[Al+3] | |
Details | Computed by OEChem 2.3.0 (PubChem release 2021.05.07) | |
Source | PubChem | |
URL | https://pubchem.ncbi.nlm.nih.gov | |
Description | Data deposited in or computed by PubChem | |
Molecular Formula |
Al2MgO4 | |
Details | Computed by PubChem 2.1 (PubChem release 2021.05.07) | |
Source | PubChem | |
URL | https://pubchem.ncbi.nlm.nih.gov | |
Description | Data deposited in or computed by PubChem | |
Molecular Weight |
142.27 g/mol | |
Details | Computed by PubChem 2.1 (PubChem release 2021.05.07) | |
Source | PubChem | |
URL | https://pubchem.ncbi.nlm.nih.gov | |
Description | Data deposited in or computed by PubChem | |
CAS No. |
12068-51-8, 11137-98-7 | |
Record name | Aluminate (AlO21-), magnesium (2:1) | |
Source | ChemIDplus | |
URL | https://pubchem.ncbi.nlm.nih.gov/substance/?source=chemidplus&sourceid=0012068518 | |
Description | ChemIDplus is a free, web search system that provides access to the structure and nomenclature authority files used for the identification of chemical substances cited in National Library of Medicine (NLM) databases, including the TOXNET system. | |
Record name | MAGNESIUM ALUMINATE | |
Source | FDA Global Substance Registration System (GSRS) | |
URL | https://gsrs.ncats.nih.gov/ginas/app/beta/substances/PAJ0L824IZ | |
Description | The FDA Global Substance Registration System (GSRS) enables the efficient and accurate exchange of information on what substances are in regulated products. Instead of relying on names, which vary across regulatory domains, countries, and regions, the GSRS knowledge base makes it possible for substances to be defined by standardized, scientific descriptions. | |
Explanation | Unless otherwise noted, the contents of the FDA website (www.fda.gov), both text and graphics, are not copyrighted. They are in the public domain and may be republished, reprinted and otherwise used freely by anyone without the need to obtain permission from FDA. Credit to the U.S. Food and Drug Administration as the source is appreciated but not required. | |
Avertissement et informations sur les produits de recherche in vitro
Veuillez noter que tous les articles et informations sur les produits présentés sur BenchChem sont destinés uniquement à des fins informatives. Les produits disponibles à l'achat sur BenchChem sont spécifiquement conçus pour des études in vitro, qui sont réalisées en dehors des organismes vivants. Les études in vitro, dérivées du terme latin "in verre", impliquent des expériences réalisées dans des environnements de laboratoire contrôlés à l'aide de cellules ou de tissus. Il est important de noter que ces produits ne sont pas classés comme médicaments et n'ont pas reçu l'approbation de la FDA pour la prévention, le traitement ou la guérison de toute condition médicale, affection ou maladie. Nous devons souligner que toute forme d'introduction corporelle de ces produits chez les humains ou les animaux est strictement interdite par la loi. Il est essentiel de respecter ces directives pour assurer la conformité aux normes légales et éthiques en matière de recherche et d'expérimentation.