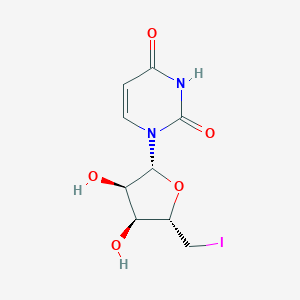
5'-Deoxy-5'-iodouridine
Vue d'ensemble
Description
5’-Deoxy-5’-iodouridine, also known as 5-Iodo-2’-deoxyuridine (5-IUdR; IdUrd), is a pyrimidine analog . It’s a halogenated thymidine that has antiviral activity against vaccinia virus (orthopoxviruses) . It is used as a radiosensitizer in human cancer studies .
Synthesis Analysis
The synthesis of three types of diphosphate analogues of 5-iodo-2’-deoxyuridine-5’-diphosphate is reported . Routes are described to the 5’-phosphonoacetamido, the 5’- N -phosphonosulfamoyl and the 5’- O -sulfamoylcarbamoyl derivatives, 2, 3 and 4 starting from 5-iodo-2’-deoxyuridine (IDU) .Molecular Structure Analysis
The molecular formula of 5’-Deoxy-5’-iodouridine is C9H11IN2O5 . The InChI code is 1S/C9H11IN2O5/c10-3-4-6(14)7(15)8(17-4)12-2-1-5(13)11-9(12)16/h1-2,4,6-8,14-15H,3H2,(H,11,13,16)/t4-,6?,7?,8-/m1/s1 .Chemical Reactions Analysis
There is limited information available on the chemical reactions involving 5’-Deoxy-5’-iodouridine .Physical And Chemical Properties Analysis
5’-Deoxy-5’-iodouridine has a molecular weight of 354.1 . The recommended storage temperature is 4°C .Applications De Recherche Scientifique
Antiviral Activity
5’-Deoxy-5’-iodouridine, also known as 5-Iodo-2’-deoxyuridine or IdUrd, has been found to have antiviral activity against vaccinia virus, a type of orthopoxvirus . This makes it a potential candidate for the treatment of diseases caused by these viruses.
Radiosensitization in Cancer Studies
IdUrd is used as a radiosensitizer in human cancer studies . Radiosensitizers are drugs that make tumor cells more sensitive to radiation therapy, thereby enhancing the effectiveness of the treatment.
Molecular Combing Assay
5’-Deoxy-5’-iodouridine has been used in molecular combing assays or DNA fiber assays . These assays are used to visualize and analyze DNA replication at the single-molecule level, providing valuable insights into the dynamics of DNA replication.
Inhibition of DNA Viral Replication
5’-Deoxy-5’-iodouridine can prevent in vitro DNA viral replication . This has been observed in herpesviruses and poxviruses, suggesting its potential use in the treatment of infections caused by these viruses.
Topical Applications for Epithelial Infections
5’-Deoxy-5’-iodouridine, when used in topical applications, is effective against epithelial infections . This makes it a potential therapeutic agent for treating skin and mucous membrane infections.
Research into Teratogenic, Tumor-Promoting, Mutagenic, and Immunosuppressive Properties
5’-Deoxy-5’-iodouridine might possess teratogenic, tumor-promoting, mutagenic, and immunosuppressive properties . This makes it a valuable compound for research into these areas, potentially leading to new insights and treatments.
Mécanisme D'action
Target of Action
5’-Deoxy-5’-iodouridine, also known as Idoxuridine, is a pyrimidine analog antiviral . Its primary target is the DNA of viruses, particularly the herpes simplex virus . It closely approximates the configuration of thymidine, one of the four building blocks of DNA .
Mode of Action
5’-Deoxy-5’-iodouridine acts as an antiviral agent by inhibiting viral replication . It substitutes itself for thymidine in viral DNA, which in turn inhibits thymidylate phosphorylase and viral DNA polymerases from properly functioning . This substitution results in the production of faulty DNA, which cannot infect or destroy tissue .
Biochemical Pathways
The primary biochemical pathway affected by 5’-Deoxy-5’-iodouridine is the DNA synthesis pathway of viruses . By substituting itself for thymidine in the DNA replication process, it disrupts the normal functioning of the pathway, leading to the production of faulty viral DNA .
Result of Action
The result of 5’-Deoxy-5’-iodouridine’s action is the inhibition of viral replication . The production of faulty DNA results in a pseudostructure which cannot infect or destroy tissue . In essence, it destroys the infective and destructive capacity of the viral material .
Safety and Hazards
Orientations Futures
Research on 5’-Deoxy-5’-iodouridine and related compounds continues, particularly in the context of their antiviral and antineoplastic activities . For example, recent research has focused on the design and synthesis of various 5’-Deoxy-5’- (4-Substituted-1,2,3-Triazol-1-yl)-Uridine Analogues as inhibitors of Mycobacterium tuberculosis Mur Ligases .
Propriétés
IUPAC Name |
1-[(2R,3R,4S,5S)-3,4-dihydroxy-5-(iodomethyl)oxolan-2-yl]pyrimidine-2,4-dione | |
---|---|---|
Source | PubChem | |
URL | https://pubchem.ncbi.nlm.nih.gov | |
Description | Data deposited in or computed by PubChem | |
InChI |
InChI=1S/C9H11IN2O5/c10-3-4-6(14)7(15)8(17-4)12-2-1-5(13)11-9(12)16/h1-2,4,6-8,14-15H,3H2,(H,11,13,16)/t4-,6-,7-,8-/m1/s1 | |
Source | PubChem | |
URL | https://pubchem.ncbi.nlm.nih.gov | |
Description | Data deposited in or computed by PubChem | |
InChI Key |
NEMNIUYGXIQPPK-XVFCMESISA-N | |
Source | PubChem | |
URL | https://pubchem.ncbi.nlm.nih.gov | |
Description | Data deposited in or computed by PubChem | |
Canonical SMILES |
C1=CN(C(=O)NC1=O)C2C(C(C(O2)CI)O)O | |
Source | PubChem | |
URL | https://pubchem.ncbi.nlm.nih.gov | |
Description | Data deposited in or computed by PubChem | |
Isomeric SMILES |
C1=CN(C(=O)NC1=O)[C@H]2[C@@H]([C@@H]([C@H](O2)CI)O)O | |
Source | PubChem | |
URL | https://pubchem.ncbi.nlm.nih.gov | |
Description | Data deposited in or computed by PubChem | |
Molecular Formula |
C9H11IN2O5 | |
Source | PubChem | |
URL | https://pubchem.ncbi.nlm.nih.gov | |
Description | Data deposited in or computed by PubChem | |
DSSTOX Substance ID |
DTXSID40162095 | |
Record name | 5'-Deoxy-5'-iodouridine | |
Source | EPA DSSTox | |
URL | https://comptox.epa.gov/dashboard/DTXSID40162095 | |
Description | DSSTox provides a high quality public chemistry resource for supporting improved predictive toxicology. | |
Molecular Weight |
354.10 g/mol | |
Source | PubChem | |
URL | https://pubchem.ncbi.nlm.nih.gov | |
Description | Data deposited in or computed by PubChem | |
CAS RN |
14259-58-6 | |
Record name | 5′-Deoxy-5′-iodouridine | |
Source | CAS Common Chemistry | |
URL | https://commonchemistry.cas.org/detail?cas_rn=14259-58-6 | |
Description | CAS Common Chemistry is an open community resource for accessing chemical information. Nearly 500,000 chemical substances from CAS REGISTRY cover areas of community interest, including common and frequently regulated chemicals, and those relevant to high school and undergraduate chemistry classes. This chemical information, curated by our expert scientists, is provided in alignment with our mission as a division of the American Chemical Society. | |
Explanation | The data from CAS Common Chemistry is provided under a CC-BY-NC 4.0 license, unless otherwise stated. | |
Record name | 5'-Deoxy-5'-iodouridine | |
Source | ChemIDplus | |
URL | https://pubchem.ncbi.nlm.nih.gov/substance/?source=chemidplus&sourceid=0014259586 | |
Description | ChemIDplus is a free, web search system that provides access to the structure and nomenclature authority files used for the identification of chemical substances cited in National Library of Medicine (NLM) databases, including the TOXNET system. | |
Record name | 5'-Deoxy-5'-iodouridine | |
Source | EPA DSSTox | |
URL | https://comptox.epa.gov/dashboard/DTXSID40162095 | |
Description | DSSTox provides a high quality public chemistry resource for supporting improved predictive toxicology. | |
Record name | Uridine, 5'-deoxy-5'-iodo | |
Source | European Chemicals Agency (ECHA) | |
URL | https://echa.europa.eu/information-on-chemicals | |
Description | The European Chemicals Agency (ECHA) is an agency of the European Union which is the driving force among regulatory authorities in implementing the EU's groundbreaking chemicals legislation for the benefit of human health and the environment as well as for innovation and competitiveness. | |
Explanation | Use of the information, documents and data from the ECHA website is subject to the terms and conditions of this Legal Notice, and subject to other binding limitations provided for under applicable law, the information, documents and data made available on the ECHA website may be reproduced, distributed and/or used, totally or in part, for non-commercial purposes provided that ECHA is acknowledged as the source: "Source: European Chemicals Agency, http://echa.europa.eu/". Such acknowledgement must be included in each copy of the material. ECHA permits and encourages organisations and individuals to create links to the ECHA website under the following cumulative conditions: Links can only be made to webpages that provide a link to the Legal Notice page. | |
Retrosynthesis Analysis
AI-Powered Synthesis Planning: Our tool employs the Template_relevance Pistachio, Template_relevance Bkms_metabolic, Template_relevance Pistachio_ringbreaker, Template_relevance Reaxys, Template_relevance Reaxys_biocatalysis model, leveraging a vast database of chemical reactions to predict feasible synthetic routes.
One-Step Synthesis Focus: Specifically designed for one-step synthesis, it provides concise and direct routes for your target compounds, streamlining the synthesis process.
Accurate Predictions: Utilizing the extensive PISTACHIO, BKMS_METABOLIC, PISTACHIO_RINGBREAKER, REAXYS, REAXYS_BIOCATALYSIS database, our tool offers high-accuracy predictions, reflecting the latest in chemical research and data.
Strategy Settings
Precursor scoring | Relevance Heuristic |
---|---|
Min. plausibility | 0.01 |
Model | Template_relevance |
Template Set | Pistachio/Bkms_metabolic/Pistachio_ringbreaker/Reaxys/Reaxys_biocatalysis |
Top-N result to add to graph | 6 |
Feasible Synthetic Routes
Q & A
Q1: Can lithium hexamethyldisilazide (LiHMDS) directly lithiate 5'-deoxy-5'-iodouridine at the C-6 position?
A1: No, direct lithiation of 5'-deoxy-5'-iodouridine at the C-6 position using LiHMDS is not observed. Research indicates that LiHMDS alone cannot generate the C-6 lithiated species. Instead, temporary silylation at either the O-4 or N-3 position of the uracil ring is crucial for successful C-6 lithiation with LiHMDS. This temporary modification likely alters the electronic properties of the uracil ring, enabling subsequent lithiation. []
Q2: What is a notable application of this temporary silylation-assisted lithiation strategy?
A2: This strategy allows for efficient intramolecular alkylation of 5'-deoxy-5'-iodouridine. Researchers successfully utilized this method to synthesize 6,5'-C-cyclouridine, a compound with potential biological activity. [] This highlights the utility of this approach for generating modified nucleosides, which could be valuable for various applications, including drug discovery.
Avertissement et informations sur les produits de recherche in vitro
Veuillez noter que tous les articles et informations sur les produits présentés sur BenchChem sont destinés uniquement à des fins informatives. Les produits disponibles à l'achat sur BenchChem sont spécifiquement conçus pour des études in vitro, qui sont réalisées en dehors des organismes vivants. Les études in vitro, dérivées du terme latin "in verre", impliquent des expériences réalisées dans des environnements de laboratoire contrôlés à l'aide de cellules ou de tissus. Il est important de noter que ces produits ne sont pas classés comme médicaments et n'ont pas reçu l'approbation de la FDA pour la prévention, le traitement ou la guérison de toute condition médicale, affection ou maladie. Nous devons souligner que toute forme d'introduction corporelle de ces produits chez les humains ou les animaux est strictement interdite par la loi. Il est essentiel de respecter ces directives pour assurer la conformité aux normes légales et éthiques en matière de recherche et d'expérimentation.