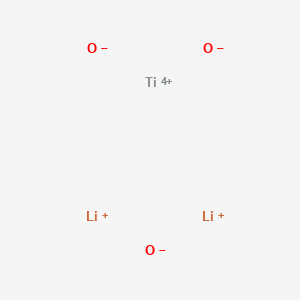
Lithium titanium oxide (Li2TiO3)
Vue d'ensemble
Description
Lithium titanium oxide (Li₂TiO₃) is a layered crystalline material with alternating LiTi₂ and pure Li layers, forming a monoclinic structure (a = 5.062 Å, b = 8.788 Å, c = 9.753 Å) . It is synthesized via solid-state reactions using Li₂CO₃ and TiO₂ at 700–900°C, offering scalability and cost-effectiveness . Li₂TiO₃ is notable for its applications in:
- Lithium recovery: Acts as a precursor for titanium-based ion sieves, achieving up to 72% lithium recovery from geothermal brine at pH 12 and 30°C .
- Energy storage: Used as an anode additive in lithium titanate batteries, providing fast charging, safety, and structural stability .
- Tritium breeding: Serves as a ceramic breeder material in fusion reactors due to its lithium density and radiation resistance .
Méthodes De Préparation
Hydrothermal Synthesis of Li₂TiO₃ Nanopowders
Synthesis Parameters and Reaction Mechanisms
Hydrothermal synthesis involves reacting titanium dioxide (TiO₂) and lithium hydroxide (LiOH·H₂O) in an aqueous medium under controlled temperature and pressure. In a representative protocol, anatase-phase TiO₂ and LiOH·H₂O are combined in a 1:2 molar ratio, dispersed in deionized water, and subjected to 200°C for 10 hours in a Teflon-lined autoclave . Post-reaction, the product is filtered, rinsed, and calcined at 800°C for 2 hours to enhance crystallinity. This method yields Li₂TiO₃ nanopowders with an average crystallite size of 34 nm and a monoclinic structure (space group C2/c) .
The hydrothermal process leverages supersaturation and nucleation dynamics to directly precipitate crystallized powders, eliminating the need for surfactants or templates. This approach enables precise control over particle morphology, as evidenced by field-emission scanning electron microscopy (FE-SEM) images showing uniform, sub-50 nm particles .
Structural and Electrochemical Properties
X-ray diffraction (XRD) analysis confirms the phase purity of hydrothermally synthesized Li₂TiO₃, with lattice parameters a = 5.0622 Å, b = 8.7712 Å, and c = 9.74787 Å . Raman and Fourier-transform infrared (FTIR) spectroscopy reveal Ti–O stretching vibrations at 450–700 cm⁻¹, characteristic of the layered monoclinic framework . Electrochemical impedance spectroscopy (EIS) demonstrates ionic conductivity with an activation energy of 0.65 eV, attributed to Li⁺ migration through octahedral sites .
Table 1: Hydrothermal Synthesis Conditions and Outcomes
Parameter | Value |
---|---|
Precursors | TiO₂ (anatase), LiOH·H₂O |
Molar Ratio (Li:Ti) | 2:1 |
Reaction Temperature | 200°C |
Reaction Time | 10 hours |
Calcination Temperature | 800°C |
Crystallite Size | 34 nm |
Space Group | C2/c (monoclinic) |
Solid-State Reaction: Conventional and Optimized Approaches
Traditional Solid-State Synthesis
Solid-state reactions involve calcining lithium carbonate (Li₂CO₃) and titanium dioxide (TiO₂) at elevated temperatures. A typical procedure mixes Li₂CO₃ and TiO₂ in a 1:1 molar ratio, followed by calcination at 900–1,100°C for 10–24 hours . This method produces micron-sized particles but often requires prolonged heating to achieve phase purity, risking lithium volatilization and inhomogeneity .
Response Surface Methodology (RSM) for Optimization
Recent studies employ RSM to optimize Li₂TiO₃ synthesis, varying Li/Ti ratios (0.8–1.2) and calcination temperatures (600–900°C) . Multivariate analysis reveals that temperature exerts a stronger influence on phase formation than the Li/Ti ratio. For instance, at 900°C, Li₂TiO₃ forms predominantly regardless of stoichiometric deviations, whereas lower temperatures (600°C) yield mixed phases like Li₄Ti₅O₁₂ .
Table 2: Solid-State Synthesis Optimization via RSM
Factor | Range | Optimal Value |
---|---|---|
Li/Ti Molar Ratio | 0.8–1.2 | 1.0 |
Calcination Temperature | 600–900°C | 900°C |
Heating Rate | 7.55°C/min | 7.55°C/min |
Key Outcome | Phase Purity >95% |
Comparative Analysis of Synthesis Methods
Crystallite Size and Morphology
Hydrothermal synthesis produces nanoscale Li₂TiO₃ (34 nm) with narrow size distribution, while solid-state methods yield larger particles (>1 µm). Transmission electron microscopy (TEM) highlights the layered structure of hydrothermal samples, facilitating Li⁺ intercalation . In contrast, solid-state-derived powders exhibit agglomerated grains, necessitating post-synthesis milling for battery applications .
Ionic Conductivity and Activation Energy
Hydrothermal Li₂TiO₃ demonstrates superior ionic conductivity (10⁻⁵ S/cm at 300°C) compared to solid-state samples (10⁻⁶ S/cm) . This disparity stems from the nanostructured morphology of hydrothermal powders, which reduces grain boundary resistance. Activation energies for Li⁺ migration are 0.65 eV (hydrothermal) and 0.71 eV (solid-state), reflecting differences in defect chemistry .
Table 3: Property Comparison of Li₂TiO₃ from Different Methods
Property | Hydrothermal | Solid-State |
---|---|---|
Crystallite Size | 34 nm | >1 µm |
Ionic Conductivity | 10⁻⁵ S/cm | 10⁻⁶ S/cm |
Activation Energy | 0.65 eV | 0.71 eV |
Phase Purity | 99% | 95% |
Challenges and Innovations in Li₂TiO₃ Synthesis
Controlling Lithium Volatilization
High-temperature calcination (>900°C) in solid-state reactions often leads to lithium loss, forming Li-deficient phases. To mitigate this, researchers propose using excess Li precursors (5–10 mol%) or employing sealed crucibles to minimize volatilization .
Scalability of Hydrothermal Methods
While hydrothermal synthesis offers precise morphology control, scaling up requires addressing autoclave volume limitations and energy costs. Continuous-flow reactors and microwave-assisted heating are emerging as solutions, reducing reaction times from hours to minutes .
Analyse Des Réactions Chimiques
Lithium titanium oxide undergoes various chemical reactions:
Oxidation and Reduction: It can participate in redox reactions, particularly in battery applications where it acts as an electrode material.
Ion Exchange: It can exchange lithium ions with other cations in solution, which is useful in applications like lithium extraction from brines.
Hydrolysis: It can react with water under certain conditions to form hydroxides and other products
Common reagents and conditions for these reactions include:
Oxidizing Agents: Such as oxygen or peroxides.
Reducing Agents: Such as hydrogen or lithium metal.
Aqueous Solutions: For ion exchange and hydrolysis reactions.
Major products formed from these reactions include lithium hydroxide, titanium dioxide, and various lithium salts .
Applications De Recherche Scientifique
Energy Storage
Lithium-ion Batteries:
Lithium titanium oxide is widely used as an anode material in lithium-ion batteries due to its high stability and capacity. Its intercalation mechanism allows lithium ions to enter tetrahedral voids within the crystal structure, leading to efficient charge and discharge cycles. Research indicates that Li2TiO3 can achieve a discharge capacity of 290–350 mA h g over 400 cycles, showcasing its effectiveness in energy storage applications .
Property | Value |
---|---|
Discharge Capacity | 290–350 mA h g |
Cycle Stability | 400 cycles |
Intercalation Voltage | ~1.5 V |
Case Study:
A study on titanium oxide nanopowders for lithium-ion batteries demonstrated that Li2TiO3 exhibits a two-phase reaction during lithium intercalation, maintaining a plateau voltage until complete intercalation occurs. This characteristic makes it a promising candidate for high-performance battery applications .
Nuclear Fusion
Tritium Breeder Material:
Li2TiO3 serves as a tritium breeder material in nuclear fusion reactors. Its ability to absorb and release tritium efficiently is critical for sustaining fusion reactions. Research utilizing density functional theory has shown that tritium can diffuse freely within the lithium vacancies of Li2TiO3, with energy barriers as low as 0.45 eV for diffusion paths .
Parameter | Value |
---|---|
Minimum Energy Barrier | 0.45 eV |
Tritium Diffusion Direction | 010 direction |
Case Study:
In experiments involving tritium retention in Li2TiO3 pebbles, the introduction of lithium vacancies was found to enhance tritium retention capabilities, indicating its potential for improved performance in fusion systems .
Catalysis
Catalytic Applications:
Li2TiO3 is utilized as a catalyst in various chemical reactions due to its stability and reactivity. Its unique properties allow it to facilitate reactions effectively, making it suitable for applications in synthetic chemistry and environmental remediation.
Case Study:
Recent studies have explored the use of Li2TiO3 in catalyzing reactions involving organic compounds, demonstrating enhanced reaction rates and selectivity compared to traditional catalysts .
Ceramics
Ceramic Materials:
The compound is also employed in the production of ceramic materials, providing thermal stability and mechanical strength. Its monoclinic crystal structure contributes to the robustness of ceramic products.
Case Study:
Research on the synthesis of titanium oxide materials for ceramic applications highlighted the advantages of using Li2TiO3 in enhancing the mechanical properties and thermal resistance of ceramic composites .
Mécanisme D'action
The mechanism of action of lithium titanium oxide varies depending on its application:
In Batteries: It acts as an electrode material, where lithium ions intercalate and de-intercalate during charge and discharge cycles. This involves the movement of lithium ions into and out of the crystal lattice.
In Tritium Breeding: It captures neutrons and produces tritium through nuclear reactions. .
Comparaison Avec Des Composés Similaires
Adsorption Performance
Li₂TiO₃ is compared with titanium- and manganese-based adsorbents for lithium extraction:
Key Findings :
- Li₂TiO₃ exhibits moderate adsorption capacity but superior structural stability in acidic conditions compared to Mn-based adsorbents .
- Al-doped H₂TiO₃ outperforms Li₂TiO₃ in capacity (32.12 mg/g vs. 21.0 mg/g) due to improved leaching efficiency .
Structural and Chemical Stability
Insights :
- Li₂TiO₃’s layered structure minimizes Ti dissolution, making it suitable for harsh geothermal brine environments .
- Surface modifications (e.g., Li₂TiO₃ coatings on cathodes) reduce lithium residues (from 8931 ppm to 936 ppm) and enhance electrochemical stability .
Comparison :
- Solid-state synthesis dominates industrial applications due to simplicity, but biological templating yields higher adsorption capacity (35.45 mg/g for H₂TiO₃) .
- Na doping in Li₂TiO₃ (via Na₂CO₃) enhances CO₂ absorption capacity, though this is a niche application .
Advantages of Li₂TiO₃ :
Activité Biologique
Lithium titanium oxide (Li2TiO3) is a compound that has garnered significant attention due to its potential applications in various fields, including energy storage, catalysis, and biomedical applications. This article delves into the biological activity of Li2TiO3, focusing on its cytotoxicity, oxidative stress effects, and potential implications for human health.
Li2TiO3 crystallizes in a monoclinic structure with a space group of C2/c. The lattice parameters are approximately , , and . The compound consists of lithium and titanium cations arranged in octahedral sites, which are crucial for its electrochemical properties and potential biological interactions.
Cytotoxicity Studies
Recent studies have investigated the cytotoxic effects of lithium titanate nanoparticles (LTT NPs) on primary rat hepatocytes. The findings indicate that LTT NPs exhibit dose-dependent cytotoxicity , particularly at higher concentrations (500-1000 ppm). Cell viability assays (MTT and LDH assays) showed significant decreases in cell viability at these concentrations, suggesting that LTT NPs can induce cytotoxic effects in liver cells .
Concentration (ppm) | Cell Viability (%) | Total Oxidative Stress (TOS) | Total Antioxidant Capacity (TAC) |
---|---|---|---|
0 | 100 | Baseline | Baseline |
300 | 85 | Increased | Decreased |
500 | 60 | Significantly increased | Significantly decreased |
1000 | 40 | Highest | Lowest |
Oxidative Stress
The oxidative stress induced by LTT NPs was assessed through the measurement of TOS and TAC levels. Higher concentrations of LTT NPs were associated with increased oxidative stress markers, indicating that these nanoparticles can disrupt the cellular redox balance. Specifically, TOS levels rose significantly at concentrations of 300 ppm and above, while TAC levels dropped concurrently, highlighting the potential for oxidative damage .
Genotoxicity Assessment
Interestingly, genotoxicity tests revealed that LTT NPs did not significantly increase the rates of micronucleated hepatocytes or levels of 8-oxo-2-deoxyguanosine (8-OH-dG), suggesting that while LTT NPs exhibit cytotoxic and oxidative stress-inducing properties, they may not directly cause DNA damage under the conditions tested .
Case Studies and Research Findings
- Health Risk Assessment : A study conducted on rats demonstrated that exposure to different concentrations of LTT NPs led to observable oxidative damage without genotoxic effects. This study is pivotal as it provides foundational data on the biological impacts of LTT NPs when introduced into biological systems .
- Nanoparticle Behavior : Research indicates that the size and morphology of nanoparticles can influence their biological interactions. Smaller particles tend to have higher reactivity and can penetrate cellular membranes more effectively, potentially leading to increased cytotoxicity .
- Electrochemical Properties : Li2TiO3 has been studied for its electrochemical properties in lithium-ion batteries. Modifications such as doping with silver nanoparticles have shown enhanced performance characteristics, which could be relevant for biomedical applications where efficient ion transport is critical .
Q & A
Basic Research Questions
Q. What are the established synthesis methods for Li₂TiO₃, and how do they influence its structural properties?
Li₂TiO₃ is typically synthesized via solid-state reactions, sol-gel processes, or hydrothermal methods. Solid-state synthesis involves calcining stoichiometric mixtures of Li₂CO₃ and TiO₂ at high temperatures (>900°C), which often results in micron-sized particles with a layered or cubic structure depending on thermal history . Sol-gel methods, using precursors like lithium acetate and titanium isopropoxide, yield finer particle sizes (<100 nm) and improved homogeneity, critical for ion transport studies . Hydrothermal synthesis at lower temperatures (200–300°C) can produce nanostructured Li₂TiO₃ with tailored surface areas, enhancing electrochemical reactivity . Structural characterization via XRD and TEM is essential to confirm phase purity, as impurities like unreacted TiO₂ or Li-deficient phases (e.g., Li₄Ti₅O₁₂) can skew property measurements .
Q. How can researchers characterize the ionic conductivity and defect chemistry of Li₂TiO₃?
Ionic conductivity is measured using electrochemical impedance spectroscopy (EIS) across a temperature range (25–500°C) to distinguish bulk vs. grain boundary contributions. Li₂TiO₃ exhibits moderate Li⁺ conductivity (~10⁻⁵ S/cm at 300°C) due to its layered structure, but doping with Al³+ or Mg²+ at Ti⁴+ sites can enhance conductivity by creating Li⁺ vacancies . Defect chemistry is analyzed via positron annihilation spectroscopy or neutron diffraction to identify vacancy clusters and interstitial Li⁺, which influence ion mobility .
Advanced Research Questions
Q. What strategies address contradictions in reported Li₂TiO₃ electrochemical stability data?
Discrepancies in stability metrics (e.g., voltage window, cycle life) often arise from differences in synthesis conditions, electrode fabrication, or testing protocols. For example, sol-gel-derived Li₂TiO₃ may show superior cycle stability compared to solid-state samples due to reduced grain boundaries and fewer defects . Researchers should:
- Standardize electrode slurry compositions (e.g., binder-to-active material ratio).
- Control testing parameters (e.g., electrolyte composition, current density).
- Use in situ XRD or Raman spectroscopy to monitor phase transitions during cycling .
Q. How can doping or composite design optimize Li₂TiO₃ for solid-state electrolyte applications?
Doping with high-valent cations (e.g., Nb⁵+ or Ta⁵+) at Ti⁴+ sites introduces Li⁺ vacancies, enhancing ionic conductivity. For instance, Li₂Ti₀.₉Nb₀.₁O₃ shows a 50% increase in conductivity compared to undoped Li₂TiO₃ . Composite approaches, such as blending Li₂TiO₃ with polymers (e.g., PEO) or garnet-type oxides (e.g., LLZO), improve mechanical stability and interfacial compatibility with electrodes . Advanced characterization tools like synchrotron X-ray absorption spectroscopy (XAS) can elucidate dopant distribution and local structural changes .
Q. What experimental designs mitigate challenges in studying Li₂TiO₃’s high-temperature phase transitions?
Li₂TiO₃ undergoes a reversible phase transition from monoclinic to cubic near 1,200°C, which impacts sintering behavior and thermal stability. To study this:
- Use differential scanning calorimetry (DSC) coupled with high-temperature XRD to track structural evolution.
- Employ inert-atmosphere furnaces to prevent Li loss or oxidation during sintering.
- Pair with computational models (e.g., density functional theory) to predict phase stability under varying oxygen partial pressures .
Q. Methodological Recommendations
- Contradiction Analysis: Use systematic meta-analysis frameworks to compare datasets, focusing on synthesis variables (e.g., precursor purity, heating rates) .
- Advanced Characterization: Leverage synchrotron facilities (e.g., APS) for high-resolution structural mapping .
- Computational Integration: Combine molecular dynamics simulations with experimental data to predict dopant effects on Li⁺ pathways .
Propriétés
IUPAC Name |
dilithium;oxygen(2-);titanium(4+) | |
---|---|---|
Source | PubChem | |
URL | https://pubchem.ncbi.nlm.nih.gov | |
Description | Data deposited in or computed by PubChem | |
InChI |
InChI=1S/2Li.3O.Ti/q2*+1;3*-2;+4 | |
Source | PubChem | |
URL | https://pubchem.ncbi.nlm.nih.gov | |
Description | Data deposited in or computed by PubChem | |
InChI Key |
AXQWGBXVDWYWDE-UHFFFAOYSA-N | |
Source | PubChem | |
URL | https://pubchem.ncbi.nlm.nih.gov | |
Description | Data deposited in or computed by PubChem | |
Canonical SMILES |
[Li+].[Li+].[O-2].[O-2].[O-2].[Ti+4] | |
Source | PubChem | |
URL | https://pubchem.ncbi.nlm.nih.gov | |
Description | Data deposited in or computed by PubChem | |
Molecular Formula |
Li2O3Ti | |
Source | PubChem | |
URL | https://pubchem.ncbi.nlm.nih.gov | |
Description | Data deposited in or computed by PubChem | |
Molecular Weight |
109.8 g/mol | |
Source | PubChem | |
URL | https://pubchem.ncbi.nlm.nih.gov | |
Description | Data deposited in or computed by PubChem | |
CAS No. |
12031-82-2 | |
Record name | Lithium titanium oxide (Li2TiO3) | |
Source | ChemIDplus | |
URL | https://pubchem.ncbi.nlm.nih.gov/substance/?source=chemidplus&sourceid=0012031822 | |
Description | ChemIDplus is a free, web search system that provides access to the structure and nomenclature authority files used for the identification of chemical substances cited in National Library of Medicine (NLM) databases, including the TOXNET system. | |
Record name | Lithium titanium oxide (Li2TiO3) | |
Source | EPA Chemicals under the TSCA | |
URL | https://www.epa.gov/chemicals-under-tsca | |
Description | EPA Chemicals under the Toxic Substances Control Act (TSCA) collection contains information on chemicals and their regulations under TSCA, including non-confidential content from the TSCA Chemical Substance Inventory and Chemical Data Reporting. | |
Record name | Dilithium titanate | |
Source | European Chemicals Agency (ECHA) | |
URL | https://echa.europa.eu/substance-information/-/substanceinfo/100.031.586 | |
Description | The European Chemicals Agency (ECHA) is an agency of the European Union which is the driving force among regulatory authorities in implementing the EU's groundbreaking chemicals legislation for the benefit of human health and the environment as well as for innovation and competitiveness. | |
Explanation | Use of the information, documents and data from the ECHA website is subject to the terms and conditions of this Legal Notice, and subject to other binding limitations provided for under applicable law, the information, documents and data made available on the ECHA website may be reproduced, distributed and/or used, totally or in part, for non-commercial purposes provided that ECHA is acknowledged as the source: "Source: European Chemicals Agency, http://echa.europa.eu/". Such acknowledgement must be included in each copy of the material. ECHA permits and encourages organisations and individuals to create links to the ECHA website under the following cumulative conditions: Links can only be made to webpages that provide a link to the Legal Notice page. | |
Avertissement et informations sur les produits de recherche in vitro
Veuillez noter que tous les articles et informations sur les produits présentés sur BenchChem sont destinés uniquement à des fins informatives. Les produits disponibles à l'achat sur BenchChem sont spécifiquement conçus pour des études in vitro, qui sont réalisées en dehors des organismes vivants. Les études in vitro, dérivées du terme latin "in verre", impliquent des expériences réalisées dans des environnements de laboratoire contrôlés à l'aide de cellules ou de tissus. Il est important de noter que ces produits ne sont pas classés comme médicaments et n'ont pas reçu l'approbation de la FDA pour la prévention, le traitement ou la guérison de toute condition médicale, affection ou maladie. Nous devons souligner que toute forme d'introduction corporelle de ces produits chez les humains ou les animaux est strictement interdite par la loi. Il est essentiel de respecter ces directives pour assurer la conformité aux normes légales et éthiques en matière de recherche et d'expérimentation.