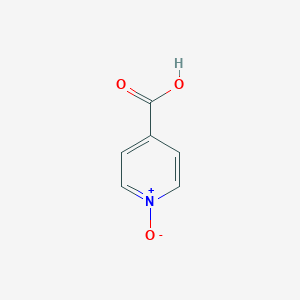
N-oxyde d'acide isonicotinique
Vue d'ensemble
Description
Applications De Recherche Scientifique
Isonicotinic acid N-oxide has a wide range of applications in scientific research:
Chemistry: It is used as a ligand in coordination chemistry to form complexes with metals
Biology: The compound is investigated for its potential as an antimicrobial agent. It has shown activity against various bacterial strains, making it a candidate for developing new antibiotics.
Medicine: Isonicotinic acid N-oxide derivatives are explored for their potential therapeutic effects, including anti-inflammatory and anticancer properties.
Industry: The compound is used in the synthesis of advanced materials, such as metal-organic frameworks (MOFs), which have applications in gas storage, separation, and catalysis.
Mécanisme D'action
Target of Action
Isonicotinic acid N-oxide primarily targets 3d metal (II) perchlorates . It interacts with these targets in ethanol-triethyl orthoformate, leading to partial substitution of perchlorate with isonicotinate-N-oxide anionic groups . It also reacts with AgClO4 or AgBF4 to yield distinct crystalline products .
Mode of Action
The compound’s mode of action involves its interaction with its targets, leading to the formation of polymeric lanthanide (III) complexes . This interaction results in changes at the molecular level, affecting the structure and function of the targets .
Biochemical Pathways
It’s known that the compound forms complexes with certain metals, which could potentially influence various biochemical processes .
Pharmacokinetics
It’s known that the compound interacts with certain metals, which could potentially affect its bioavailability .
Result of Action
The result of isonicotinic acid N-oxide’s action is the formation of distinct crystalline products when it reacts with AgClO4 or AgBF4 . These products could potentially have various molecular and cellular effects.
Action Environment
The action, efficacy, and stability of isonicotinic acid N-oxide can be influenced by various environmental factors. For instance, the presence of certain metals in the environment can affect the compound’s ability to form complexes . .
Safety and Hazards
Orientations Futures
In search of anti-inflammatory compounds, novel scaffolds containing isonicotinic acid N-oxide motif were synthesized via an efficient strategy . The compounds were screened for their in vitro anti-inflammatory activity . Decisively, further development and fine tuning of these isonicotinates based scaffolds for the treatment of various aberrations is still a wide-open field of research .
Analyse Biochimique
Biochemical Properties
Isonicotinic acid N-oxide plays a significant role in biochemical reactions, particularly in the formation of complexes with metal ions. It interacts with enzymes and proteins, such as catalase-peroxidase enzymes, which are crucial for its activation and subsequent biochemical activity . The compound’s interaction with these enzymes often involves the formation of stable complexes that can influence the enzyme’s activity and stability.
Cellular Effects
Isonicotinic acid N-oxide affects various types of cells and cellular processes. It has been shown to influence cell signaling pathways, gene expression, and cellular metabolism. For instance, in Mycobacterium tuberculosis, isonicotinic acid N-oxide derivatives inhibit the synthesis of mycolic acids, essential components of the bacterial cell wall . This inhibition disrupts cell wall integrity, leading to cell death. Additionally, the compound can modulate gene expression by interacting with transcription factors and other regulatory proteins.
Molecular Mechanism
The molecular mechanism of isonicotinic acid N-oxide involves its activation by catalase-peroxidase enzymes, leading to the formation of reactive intermediates. These intermediates can bind to various biomolecules, including enzymes and nucleic acids, resulting in enzyme inhibition or activation and changes in gene expression . The compound’s ability to form stable complexes with metal ions also plays a crucial role in its biochemical activity.
Temporal Effects in Laboratory Settings
In laboratory settings, the effects of isonicotinic acid N-oxide can change over time due to its stability and degradation. The compound is relatively stable under standard laboratory conditions, but its activity can diminish over extended periods due to gradual degradation . Long-term studies have shown that isonicotinic acid N-oxide can have sustained effects on cellular function, particularly in in vitro and in vivo models.
Dosage Effects in Animal Models
The effects of isonicotinic acid N-oxide vary with different dosages in animal models. At lower doses, the compound can exhibit therapeutic effects, such as antimicrobial activity against Mycobacterium tuberculosis . At higher doses, it may cause toxic or adverse effects, including hepatotoxicity and neurotoxicity. These threshold effects highlight the importance of careful dosage optimization in therapeutic applications.
Metabolic Pathways
Isonicotinic acid N-oxide is involved in several metabolic pathways, including those related to its activation and detoxification. Enzymes such as N-arylaminoacetyl transferases (NATs) play a role in the acetylation of the compound, which can reduce its therapeutic effectiveness . The compound’s metabolism can also affect metabolic flux and metabolite levels, influencing overall cellular function.
Transport and Distribution
Within cells and tissues, isonicotinic acid N-oxide is transported and distributed through interactions with transporters and binding proteins. These interactions can affect the compound’s localization and accumulation, influencing its biochemical activity . The compound’s ability to form complexes with metal ions also plays a role in its transport and distribution.
Subcellular Localization
Isonicotinic acid N-oxide’s subcellular localization is influenced by targeting signals and post-translational modifications. The compound can be directed to specific compartments or organelles, such as the mitochondria or nucleus, where it exerts its biochemical effects . This localization is crucial for its activity, as it allows the compound to interact with specific biomolecules and enzymes within the cell.
Méthodes De Préparation
Synthetic Routes and Reaction Conditions: Isonicotinic acid N-oxide can be synthesized through the oxidation of isonicotinic acid. One common method involves the use of hydrogen peroxide as the oxidizing agent in the presence of a catalyst such as acetic acid. The reaction typically proceeds under mild conditions, with the temperature maintained around 60°C to 80°C.
Industrial Production Methods: In industrial settings, the production of isonicotinic acid N-oxide may involve more efficient and scalable processes. For example, the oxidation of isonicotinic acid can be carried out using stronger oxidizing agents like potassium permanganate or chromium trioxide. These methods ensure higher yields and purity of the final product.
Analyse Des Réactions Chimiques
Types of Reactions: Isonicotinic acid N-oxide undergoes various chemical reactions, including:
Oxidation: Further oxidation can lead to the formation of more complex oxides.
Reduction: Reduction reactions can convert the N-oxide group back to the original amine group.
Substitution: The compound can participate in nucleophilic substitution reactions, where the N-oxide group can be replaced by other functional groups.
Common Reagents and Conditions:
Oxidation: Hydrogen peroxide, potassium permanganate, chromium trioxide.
Reduction: Sodium borohydride, lithium aluminum hydride.
Substitution: Various nucleophiles such as amines, alcohols, and thiols.
Major Products Formed:
Oxidation: Higher oxides or complex coordination compounds.
Reduction: Isonicotinic acid or its derivatives.
Substitution: Substituted pyridine derivatives.
Comparaison Avec Des Composés Similaires
Isonicotinic acid N-oxide can be compared with other similar compounds such as:
Nicotinic acid N-oxide: Similar in structure but differs in the position of the carboxylic acid group.
Picolinic acid N-oxide: Another isomer with the carboxylic acid group at the 2-position.
Quinolinic acid N-oxide: A derivative of quinoline with an N-oxide group.
Uniqueness: Isonicotinic acid N-oxide is unique due to its specific position of the carboxylic acid group at the 4-position, which influences its chemical reactivity and biological activity. This positional difference can lead to distinct properties and applications compared to its isomers.
Propriétés
IUPAC Name |
1-oxidopyridin-1-ium-4-carboxylic acid | |
---|---|---|
Source | PubChem | |
URL | https://pubchem.ncbi.nlm.nih.gov | |
Description | Data deposited in or computed by PubChem | |
InChI |
InChI=1S/C6H5NO3/c8-6(9)5-1-3-7(10)4-2-5/h1-4H,(H,8,9) | |
Source | PubChem | |
URL | https://pubchem.ncbi.nlm.nih.gov | |
Description | Data deposited in or computed by PubChem | |
InChI Key |
QCWTWMJMLSKQCJ-UHFFFAOYSA-N | |
Source | PubChem | |
URL | https://pubchem.ncbi.nlm.nih.gov | |
Description | Data deposited in or computed by PubChem | |
Canonical SMILES |
C1=C[N+](=CC=C1C(=O)O)[O-] | |
Source | PubChem | |
URL | https://pubchem.ncbi.nlm.nih.gov | |
Description | Data deposited in or computed by PubChem | |
Molecular Formula |
C6H5NO3 | |
Source | PubChem | |
URL | https://pubchem.ncbi.nlm.nih.gov | |
Description | Data deposited in or computed by PubChem | |
DSSTOX Substance ID |
DTXSID6065557 | |
Record name | 4-Pyridinecarboxylic acid 1-oxide | |
Source | EPA DSSTox | |
URL | https://comptox.epa.gov/dashboard/DTXSID6065557 | |
Description | DSSTox provides a high quality public chemistry resource for supporting improved predictive toxicology. | |
Molecular Weight |
139.11 g/mol | |
Source | PubChem | |
URL | https://pubchem.ncbi.nlm.nih.gov | |
Description | Data deposited in or computed by PubChem | |
CAS No. |
13602-12-5 | |
Record name | Isonicotinic acid N-oxide | |
Source | CAS Common Chemistry | |
URL | https://commonchemistry.cas.org/detail?cas_rn=13602-12-5 | |
Description | CAS Common Chemistry is an open community resource for accessing chemical information. Nearly 500,000 chemical substances from CAS REGISTRY cover areas of community interest, including common and frequently regulated chemicals, and those relevant to high school and undergraduate chemistry classes. This chemical information, curated by our expert scientists, is provided in alignment with our mission as a division of the American Chemical Society. | |
Explanation | The data from CAS Common Chemistry is provided under a CC-BY-NC 4.0 license, unless otherwise stated. | |
Record name | 4-Pyridinecarboxylic acid, 1-oxide | |
Source | ChemIDplus | |
URL | https://pubchem.ncbi.nlm.nih.gov/substance/?source=chemidplus&sourceid=0013602125 | |
Description | ChemIDplus is a free, web search system that provides access to the structure and nomenclature authority files used for the identification of chemical substances cited in National Library of Medicine (NLM) databases, including the TOXNET system. | |
Record name | 13602-12-5 | |
Source | DTP/NCI | |
URL | https://dtp.cancer.gov/dtpstandard/servlet/dwindex?searchtype=NSC&outputformat=html&searchlist=63044 | |
Description | The NCI Development Therapeutics Program (DTP) provides services and resources to the academic and private-sector research communities worldwide to facilitate the discovery and development of new cancer therapeutic agents. | |
Explanation | Unless otherwise indicated, all text within NCI products is free of copyright and may be reused without our permission. Credit the National Cancer Institute as the source. | |
Record name | 4-Pyridinecarboxylic acid, 1-oxide | |
Source | EPA Chemicals under the TSCA | |
URL | https://www.epa.gov/chemicals-under-tsca | |
Description | EPA Chemicals under the Toxic Substances Control Act (TSCA) collection contains information on chemicals and their regulations under TSCA, including non-confidential content from the TSCA Chemical Substance Inventory and Chemical Data Reporting. | |
Record name | 4-Pyridinecarboxylic acid 1-oxide | |
Source | EPA DSSTox | |
URL | https://comptox.epa.gov/dashboard/DTXSID6065557 | |
Description | DSSTox provides a high quality public chemistry resource for supporting improved predictive toxicology. | |
Record name | Pyridin-N-oxide-4-carboxylic acid | |
Source | European Chemicals Agency (ECHA) | |
URL | https://echa.europa.eu/substance-information/-/substanceinfo/100.033.700 | |
Description | The European Chemicals Agency (ECHA) is an agency of the European Union which is the driving force among regulatory authorities in implementing the EU's groundbreaking chemicals legislation for the benefit of human health and the environment as well as for innovation and competitiveness. | |
Explanation | Use of the information, documents and data from the ECHA website is subject to the terms and conditions of this Legal Notice, and subject to other binding limitations provided for under applicable law, the information, documents and data made available on the ECHA website may be reproduced, distributed and/or used, totally or in part, for non-commercial purposes provided that ECHA is acknowledged as the source: "Source: European Chemicals Agency, http://echa.europa.eu/". Such acknowledgement must be included in each copy of the material. ECHA permits and encourages organisations and individuals to create links to the ECHA website under the following cumulative conditions: Links can only be made to webpages that provide a link to the Legal Notice page. | |
Retrosynthesis Analysis
AI-Powered Synthesis Planning: Our tool employs the Template_relevance Pistachio, Template_relevance Bkms_metabolic, Template_relevance Pistachio_ringbreaker, Template_relevance Reaxys, Template_relevance Reaxys_biocatalysis model, leveraging a vast database of chemical reactions to predict feasible synthetic routes.
One-Step Synthesis Focus: Specifically designed for one-step synthesis, it provides concise and direct routes for your target compounds, streamlining the synthesis process.
Accurate Predictions: Utilizing the extensive PISTACHIO, BKMS_METABOLIC, PISTACHIO_RINGBREAKER, REAXYS, REAXYS_BIOCATALYSIS database, our tool offers high-accuracy predictions, reflecting the latest in chemical research and data.
Strategy Settings
Precursor scoring | Relevance Heuristic |
---|---|
Min. plausibility | 0.01 |
Model | Template_relevance |
Template Set | Pistachio/Bkms_metabolic/Pistachio_ringbreaker/Reaxys/Reaxys_biocatalysis |
Top-N result to add to graph | 6 |
Feasible Synthetic Routes
Avertissement et informations sur les produits de recherche in vitro
Veuillez noter que tous les articles et informations sur les produits présentés sur BenchChem sont destinés uniquement à des fins informatives. Les produits disponibles à l'achat sur BenchChem sont spécifiquement conçus pour des études in vitro, qui sont réalisées en dehors des organismes vivants. Les études in vitro, dérivées du terme latin "in verre", impliquent des expériences réalisées dans des environnements de laboratoire contrôlés à l'aide de cellules ou de tissus. Il est important de noter que ces produits ne sont pas classés comme médicaments et n'ont pas reçu l'approbation de la FDA pour la prévention, le traitement ou la guérison de toute condition médicale, affection ou maladie. Nous devons souligner que toute forme d'introduction corporelle de ces produits chez les humains ou les animaux est strictement interdite par la loi. Il est essentiel de respecter ces directives pour assurer la conformité aux normes légales et éthiques en matière de recherche et d'expérimentation.