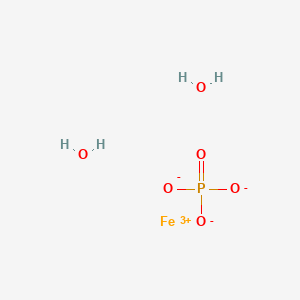
Iron(III) phosphate dihydrate
Vue d'ensemble
Description
Iron(III) phosphate dihydrate (FePO₄·2H₂O) is a hydrated inorganic compound with a molecular weight of 186.85 g/mol . It exists as a pale white powder and is sparingly soluble in water under neutral conditions, though its solubility increases significantly in the presence of phosphoric acid . The compound is structured with two water molecules coordinated to the iron-phosphate matrix, which influences its thermal stability and reactivity.
Key properties include:
Méthodes De Préparation
Direct Precipitation Using Phosphoric Acid and Metallic Iron
Reaction Mechanism and Stoichiometric Control
The direct reaction between phosphoric acid (H₃PO₄) and metallic iron powder under oxidative conditions is a widely used industrial method. As detailed in CN105895914A, a molar ratio of 2.2:1 (P:Fe) is achieved by adding 115–126 parts by weight of 85% H₃PO₄ to 400 parts deionized water, followed by 56 parts reduced iron powder at 50–65°C . Hydrogen peroxide (56–68 parts) is introduced post-ball-milling to oxidize residual Fe²⁺, ensuring complete conversion to Fe³⁺. The final spray-drying step at 150–180°C yields spherical particles with a median diameter of 15–25 μm .
Optimization of Crystallization Conditions
Elevated temperatures during the hydrothermal treatment (60–140°C for 6–12 hours) promote the formation of metastrengite (FePO₄·2H₂O) over amorphous phases. At 140°C, the crystallinity index increases to 92%, as measured by X-ray diffraction (XRD) . However, excessive H₂O₂ concentrations (>68 parts) induce particle agglomeration, reducing specific surface area from 45 m²/g to 28 m²/g .
Two-Pot Synthesis via Ferrous Phosphate Oxidation
Vivianite Intermediate Formation
The two-pot method, described in US9174846B2, involves precipitating vivianite (Fe₃(PO₄)₂·8H₂O) by mixing ferrous sulfate (FeSO₄·7H₂O) with diammonium phosphate ((NH₄)₂HPO₄) at pH 4–5 . Aging the slurry at 45–65°C for 2–4 hours enlarges particle size, facilitating filtration and reducing sulfate residues to <0.1 wt% .
Oxidation and Crystallization
The vivianite is suspended in H₃PO₄ (1–3 M) and oxidized with 30% H₂O₂ at 85–100°C. This step converts Fe²⁺ to Fe³⁺, yielding FePO₄·2H₂O with a phosphorus-to-iron ratio of 1.05–1.10 . Crystalline phase purity depends on final pH:
Decomplexation of Ferric Phosphate Complex Solutions
Complex Formation and Dilution
EP4410742NWA1 discloses a method where ferric oxide (Fe₂O₃) reacts with 35–85% H₃PO₄ at a P:Fe ratio of 2–10:1, forming a soluble ferric phosphate complex . Diluting the complex with 0.3–30 volumes of water induces decomplexation, precipitating FePO₄·2H₂O. At 60–160°C and 0.1–0.5 MPa pressure, the yield reaches 98% with a median particle size of 8–12 μm .
Recirculation of Mother Liquor
The second mother liquor from solid-liquid separation is recycled to adjust the P:Fe ratio in subsequent batches, reducing phosphoric acid consumption by 22% .
Oxidative Routes with Inorganic Agents
Sodium Chlorate-Mediated Oxidation
As reported in a scientific study, Fe₃(PO₄)₂ is oxidized using NaClO₃ at 80°C and pH 2, achieving 99% Fe³⁺ conversion . The resulting FePO₄·2H₂O exhibits a discharge capacity of 155 mAh/g when used in LiFePO₄ cathodes, outperforming H₂O₂-derived samples by 12% .
Comparative Analysis of Oxidants
Industrial Applications and Scalability
Battery-Grade Material Production
Spray-dried FePO₄·2H₂O from direct precipitation is preferred for LiFePO₄ synthesis due to its spherical morphology and high tap density (1.4–1.6 g/cm³) . Two-pot methods, while costlier, eliminate sulfate contaminants that degrade battery cycle life .
Environmental and Economic Considerations
Decomplexation routes reduce wastewater generation by 40% compared to direct precipitation . However, they require pressurized reactors, increasing capital costs by 18–25% .
Analyse Des Réactions Chimiques
Catalytic Reactions
FePO₄·2H₂O acts as a Lewis acid catalyst in organic synthesis due to its Brønsted acidity and surface-active sites:
-
Kinetic Data : Activation energy for oxidative dehydrogenation: -14.8 kJ/mol (linear range: 0–0.5 mg/L) .
-
Reusability : Retains >90% activity after 5 cycles due to structural stability .
Phase Transformation and Thermal Decomposition
Thermal treatment induces dehydration and structural rearrangement:
-
Activation Energy : Phase transformation requires 9.619 kJ/mol .
-
Structural Impact : Dehydration reduces surface area but enhances electrochemical stability for battery applications .
Complexation and Dissolution Dynamics
In aqueous systems, FePO₄·2H₂O forms soluble complexes influenced by pH:
pH Range | Dominant Species | Equilibrium Constant (log K) | Implications | Source |
---|---|---|---|---|
0.2–1.3 | , | Enhances phosphorus solubility in acidic soils. | ||
>2.5 | , | – | Precipitation dominates, reducing bioavailability. |
-
Kinetics : Tetranuclear complex forms transiently (milliseconds) before dissociating into .
-
Dissolution Rate : Amorphous FePO₄·2H₂O dissolves 3× faster than crystalline forms, critical for nutrient release in agriculture .
Environmental and Biological Interactions
Applications De Recherche Scientifique
Agricultural Applications
Molluscicide in Organic Farming
Iron(III) phosphate dihydrate is recognized as a non-toxic molluscicide, particularly effective against slugs and snails. Its use is approved in organic farming due to its safety for non-target organisms, including beneficial insects and wildlife. The compound disrupts the feeding mechanisms of these pests without posing significant risks to the environment .
Catalytic Applications
Selective Catalysis
The compound serves as a catalyst in various chemical reactions, particularly in organic synthesis. Its microporous and mesoporous structures enhance its catalytic properties by providing a larger surface area for reactions. This makes it suitable for selective catalysis, where specific reactions are favored over others .
Energy Storage
Electrode Material for Lithium Batteries
this compound is utilized as a precursor in the synthesis of lithium iron phosphate (LiFePO4), which is a prominent cathode material in lithium-ion batteries. LiFePO4 offers advantages such as thermal stability, safety, and a long cycle life, making it ideal for electric vehicles and energy storage systems .
Ceramic and Glass Industries
Ceramic Glazing
In the ceramic industry, iron phosphate dihydrate is used as an ingredient in glazes due to its ability to impart color and improve the mechanical properties of ceramic products. It contributes to the aesthetic qualities of ceramics while enhancing their durability .
Surface Treatment
Anti-Corrosive Coatings
The compound is employed in surface passivation processes to provide anti-corrosive coatings on metals. This application is crucial in industries where metal components are exposed to harsh environments, helping to prolong the lifespan of these materials .
Case Study 1: Use as Molluscicide
A study conducted on the effectiveness of this compound as a molluscicide demonstrated significant reductions in slug populations in organic farming settings. The results indicated that application rates of 1-2 g/m² effectively controlled pest populations without harming beneficial organisms.
Case Study 2: Lithium-Ion Batteries
Research on lithium iron phosphate (LiFePO4) synthesized from this compound showed promising results in battery performance tests. The study highlighted improved thermal stability and cycle life compared to conventional lithium cobalt oxide batteries, making LiFePO4 a favorable option for electric vehicle applications.
Mécanisme D'action
The mechanism of action of iron(III) phosphate dihydrate involves its ability to act as a catalyst in various chemical reactions. It can facilitate the formation of specific products by providing an active site for the reaction to occur. In biological systems, its peroxidase-like activity allows it to catalyze the breakdown of hydrogen peroxide into water and oxygen, mimicking the action of natural enzymes .
Comparaison Avec Des Composés Similaires
Other Iron Phosphates
Anhydrous Iron(III) Phosphate (FePO₄)
- Molecular Weight : 150.82 g/mol .
- Solubility : Insoluble in water but reacts with strong acids.
- Thermal Stability : Dehydrates above 450°C to form FePO₄ .
- Applications : Catalyst in organic synthesis and cathode material precursor .
Iron(III) Pyrophosphate (Fe₄(P₂O₇)₃)
- Solubility : Poor aqueous solubility, often used in fortified foods due to low reactivity .
- Applications : Dietary iron supplements .
Table 1: Comparison of Iron Phosphates
Compound | Molecular Formula | Molecular Weight (g/mol) | Solubility in Water | Key Applications |
---|---|---|---|---|
Iron(III) Phosphate Dihydrate | FePO₄·2H₂O | 186.85 | Low (pH-dependent) | Batteries, molluscicides |
Anhydrous FePO₄ | FePO₄ | 150.82 | Insoluble | Catalysts, ceramics |
Iron(III) Pyrophosphate | Fe₄(P₂O₇)₃ | 745.21 | Insoluble | Food fortification |
Other Iron Compounds
Iron(III) Chloride Hexahydrate (FeCl₃·6H₂O)
- Solubility : Highly soluble in water (920 g/L at 20°C) .
- Thermal Behavior : Loses water molecules above 37°C, forming anhydrous FeCl₃ .
- Applications : Coagulant in water treatment and etching agent .
Iron(III) Citrate (FeC₆H₅O₇)
- Solubility : Moderately soluble in water; forms complexes with organic acids .
- Applications : Dietary supplements and microbial growth media .
Table 2: Comparison with Other Iron Compounds
Compound | Solubility in Water | Thermal Stability (°C) | Key Applications |
---|---|---|---|
FePO₄·2H₂O | Low | Dehydrates at ~300°C | Energy storage, farming |
FeCl₃·6H₂O | 920 g/L | Decomposes at 37°C | Water treatment |
Iron(III) Citrate | Moderate | Stable up to 200°C | Pharmaceuticals, biology |
Other Metal Phosphate Dihydrates
Calcium Phosphate Dihydrate (CaHPO₄·2H₂O)
- Solubility: Very low (0.02 g/L at 25°C); non-hygroscopic .
- Thermal Behavior : Loses water at 109°C; used as a tableting excipient .
- Applications : Pharmaceutical tablets and dental materials .
Copper(II) Phosphate Dihydrate (Cu₃(PO₄)₂·2H₂O)
- Solubility : Insoluble in water; reacts with acids .
- Applications : Corrosion inhibition and pigments .
Table 3: Comparison with Metal Phosphate Dihydrates
Compound | Cation | Solubility in Water | Applications |
---|---|---|---|
FePO₄·2H₂O | Fe³⁺ | Low | Batteries, agriculture |
CaHPO₄·2H₂O | Ca²⁺ | 0.02 g/L | Pharmaceuticals |
Cu₃(PO₄)₂·2H₂O | Cu²⁺ | Insoluble | Industrial coatings |
Key Research Findings
Solubility Behavior : FePO₄·2H₂O’s solubility increases by 5 orders of magnitude with phosphoric acid concentration (1.13–10.7 wt%) but decreases with rising temperature (298–363 K) due to coordination species dissociation .
Thermal Decomposition : Dehydration occurs below 300°C, while desulfurization and phase transformation to trigonal FePO₄ occur at 400–550°C .
Morphological Control : Particle size and crystallinity of FePO₄·2H₂O can be tuned via mixed-acid precipitation, impacting battery performance .
Activité Biologique
Iron(III) phosphate dihydrate (FePO₄·2H₂O) is an inorganic compound that has garnered significant attention for its diverse biological activities, particularly in agriculture and catalysis. This article delves into its biological properties, synthesis methods, and applications, supported by case studies and relevant research findings.
Chemical Structure and Properties
This compound appears as an off-white powder and consists of iron in the +3 oxidation state, phosphate ions, and two water molecules per formula unit. The octahedral geometry of the iron center, influenced by the coordination of water molecules, plays a crucial role in its reactivity and biological activity.
Biological Activity
1. Molluscicidal Properties:
this compound is recognized as a molluscicide , approved for use in organic farming. It effectively disrupts the metabolism of pests such as slugs and snails without adversely affecting beneficial insects. This selective toxicity is particularly valuable in sustainable agricultural practices.
2. Nutrient Availability:
The compound also influences the bioavailability of phosphorus in soils. Its interaction with soil components can enhance phosphorus uptake by plants, thus improving growth and yield. Research indicates that the presence of this compound can alter soil chemistry, facilitating better nutrient absorption.
3. Catalytic Applications:
In addition to its agricultural uses, this compound serves as a catalyst in various chemical reactions. Notably, it has been utilized in the Biginelli reaction to synthesize 3,4-dihydropyrimidinones and thiones, which possess therapeutic properties . The compound's catalytic efficiency stems from its ability to stabilize reaction intermediates and promote favorable reaction pathways.
Synthesis Methods
This compound is typically synthesized through precipitation methods involving the reaction between iron salts and phosphoric acid in an aqueous medium. Common synthesis routes include:
-
Precipitation Reaction:
- Solvothermal Methods: These involve heating the reactants under pressure to enhance crystallinity and yield.
Case Studies
Case Study 1: Agricultural Application
A study conducted by researchers demonstrated that applying this compound at specific concentrations significantly reduced slug populations in organic farms while maintaining healthy populations of beneficial insects. This study highlights the compound's potential for integrated pest management strategies.
Case Study 2: Catalytic Efficiency
In a series of experiments focusing on the Biginelli reaction, this compound was found to facilitate high yields of dihydropyrimidinones under mild conditions. The reaction showcased excellent selectivity and efficiency, confirming the compound's utility as a green catalyst in organic synthesis .
Comparative Analysis
The following table compares this compound with other related compounds regarding their biological activity and applications:
Compound Name | Formula | Biological Activity | Applications |
---|---|---|---|
This compound | FePO₄·2H₂O | Molluscicide; enhances phosphorus availability | Agriculture; Catalysis |
Iron(II) phosphate | Fe₃(PO₄)₂ | Limited biological activity | Less soluble; environmental studies |
Lithium iron phosphate | LiFePO₄ | Used in battery technology | Energy storage |
Strengite | FePO₄·2H₂O | Similar to iron(III) phosphate but less studied | Environmental remediation |
Q & A
Basic Questions
Q. What are the laboratory synthesis methods for Iron(III) phosphate dihydrate (FePO₄·2H₂O)?
- Hydrothermal synthesis : Reacting ferrous sulfate (FeSO₄) with wet-process phosphoric acid (H₃PO₄) under controlled temperature and stirring rates, followed by oxidation with hydrogen peroxide (H₂O₂) and pH adjustment with ammonia (NH₃). The product is filtered, washed, and dried to yield FePO₄·2H₂O .
- Homogeneous co-precipitation : Mixing iron and phosphate precursors in aqueous solutions under precise stoichiometric control to precipitate amorphous FePO₄·2H₂O, which is later calcined to improve crystallinity .
Q. How is X-ray diffraction (XRD) used to characterize FePO₄·2H₂O crystallinity?
- XRD identifies the amorphous or crystalline nature of synthesized FePO₄·2H₂O. For example, synthetic FePO₄·2H₂O may exhibit amorphous characteristics in XRD patterns, while calcined samples (e.g., above 550°C) show orthorhombic or monoclinic crystalline phases .
Q. What is the correct nomenclature and chemical formula for FePO₄·2H₂O?
- The compound is systematically named This compound with the formula FePO₄·2H₂O. Its molecular weight is 186.85 g/mol, and its CAS registry number is 13463-10-0 .
Q. What analytical techniques are used to study thermal decomposition pathways?
- Thermogravimetric analysis (TGA) and differential scanning calorimetry (DSC) quantify mass loss and phase transitions. For FePO₄·2H₂O, dehydration occurs below 300°C, while desulfurization (removal of sulfate impurities) occurs between 400–500°C .
Advanced Research Questions
Q. How do temperature and phosphoric acid concentration affect FePO₄·2H₂O solubility?
- Solubility increases by 5 orders of magnitude with rising H₃PO₄ concentration (1.13–10.7 wt%) due to coordination species formation (e.g., [Fe(PO₄)₂]³⁻). Conversely, increasing temperature (298–363 K) reduces solubility by 1–2 orders of magnitude, favoring recrystallization into orthorhombic FePO₄ .
Q. What are the critical temperature thresholds for phase transformations during calcination?
- Dehydration : Complete removal of bound water occurs below 300°C.
- Desulfurization : Sulfate impurities decompose between 400–500°C.
- Phase transition : Amorphous FePO₄·2H₂O transforms into crystalline FePO₄ (orthorhombic) at 530°C, achieving full conversion after 6 hours at 550°C .
Q. How does phosphoric acid influence phase transformation mechanisms during thermal treatment?
- Excess H₃PO₄ stabilizes dissolved Fe³⁺-phosphate complexes, accelerating dissolution-recrystallization kinetics. This promotes Ostwald ripening, leading to larger crystallites and reduced surface defects in the final FePO₄ .
Q. What role does precursor morphology play in lithium iron phosphate (LiFePO₄) battery performance?
- FePO₄·2H₂O with high surface area and low sulfur content enhances LiFePO₄’s charge-discharge rates. Particle fusion during calcination above 500°C reduces surface area, increasing charge-transfer impedance .
Q. Methodological Considerations
- Synthesis Optimization : Control pH (5–6) and reaction time to minimize impurities like sulfates, which degrade electrochemical performance .
- Characterization Workflow : Combine XRD, TGA/DSC, and BET surface area analysis to correlate synthesis parameters with material properties .
- Solubility Modeling : Use empirical equations to predict FePO₄·2H₂O solubility as a function of H₃PO₄ and temperature, enabling precise control over precipitation kinetics .
Q. Conflicting Data Notes
Propriétés
IUPAC Name |
iron(3+);phosphate;dihydrate | |
---|---|---|
Source | PubChem | |
URL | https://pubchem.ncbi.nlm.nih.gov | |
Description | Data deposited in or computed by PubChem | |
InChI |
InChI=1S/Fe.H3O4P.2H2O/c;1-5(2,3)4;;/h;(H3,1,2,3,4);2*1H2/q+3;;;/p-3 | |
Source | PubChem | |
URL | https://pubchem.ncbi.nlm.nih.gov | |
Description | Data deposited in or computed by PubChem | |
InChI Key |
BMTOKWDUYJKSCN-UHFFFAOYSA-K | |
Source | PubChem | |
URL | https://pubchem.ncbi.nlm.nih.gov | |
Description | Data deposited in or computed by PubChem | |
Canonical SMILES |
O.O.[O-]P(=O)([O-])[O-].[Fe+3] | |
Source | PubChem | |
URL | https://pubchem.ncbi.nlm.nih.gov | |
Description | Data deposited in or computed by PubChem | |
Molecular Formula |
FeH4O6P | |
Source | PubChem | |
URL | https://pubchem.ncbi.nlm.nih.gov | |
Description | Data deposited in or computed by PubChem | |
DSSTOX Substance ID |
DTXSID60928695 | |
Record name | Iron(3+) phosphate--water (1/1/2) | |
Source | EPA DSSTox | |
URL | https://comptox.epa.gov/dashboard/DTXSID60928695 | |
Description | DSSTox provides a high quality public chemistry resource for supporting improved predictive toxicology. | |
Molecular Weight |
186.85 g/mol | |
Source | PubChem | |
URL | https://pubchem.ncbi.nlm.nih.gov | |
Description | Data deposited in or computed by PubChem | |
CAS No. |
13463-10-0 | |
Record name | Iron(3+) phosphate--water (1/1/2) | |
Source | EPA DSSTox | |
URL | https://comptox.epa.gov/dashboard/DTXSID60928695 | |
Description | DSSTox provides a high quality public chemistry resource for supporting improved predictive toxicology. | |
Retrosynthesis Analysis
AI-Powered Synthesis Planning: Our tool employs the Template_relevance Pistachio, Template_relevance Bkms_metabolic, Template_relevance Pistachio_ringbreaker, Template_relevance Reaxys, Template_relevance Reaxys_biocatalysis model, leveraging a vast database of chemical reactions to predict feasible synthetic routes.
One-Step Synthesis Focus: Specifically designed for one-step synthesis, it provides concise and direct routes for your target compounds, streamlining the synthesis process.
Accurate Predictions: Utilizing the extensive PISTACHIO, BKMS_METABOLIC, PISTACHIO_RINGBREAKER, REAXYS, REAXYS_BIOCATALYSIS database, our tool offers high-accuracy predictions, reflecting the latest in chemical research and data.
Strategy Settings
Precursor scoring | Relevance Heuristic |
---|---|
Min. plausibility | 0.01 |
Model | Template_relevance |
Template Set | Pistachio/Bkms_metabolic/Pistachio_ringbreaker/Reaxys/Reaxys_biocatalysis |
Top-N result to add to graph | 6 |
Feasible Synthetic Routes
Avertissement et informations sur les produits de recherche in vitro
Veuillez noter que tous les articles et informations sur les produits présentés sur BenchChem sont destinés uniquement à des fins informatives. Les produits disponibles à l'achat sur BenchChem sont spécifiquement conçus pour des études in vitro, qui sont réalisées en dehors des organismes vivants. Les études in vitro, dérivées du terme latin "in verre", impliquent des expériences réalisées dans des environnements de laboratoire contrôlés à l'aide de cellules ou de tissus. Il est important de noter que ces produits ne sont pas classés comme médicaments et n'ont pas reçu l'approbation de la FDA pour la prévention, le traitement ou la guérison de toute condition médicale, affection ou maladie. Nous devons souligner que toute forme d'introduction corporelle de ces produits chez les humains ou les animaux est strictement interdite par la loi. Il est essentiel de respecter ces directives pour assurer la conformité aux normes légales et éthiques en matière de recherche et d'expérimentation.