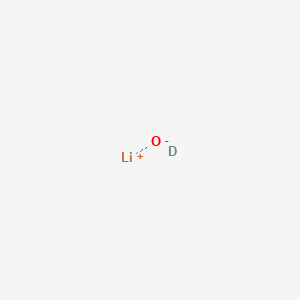
Lithium (2H)hydroxide
Vue d'ensemble
Description
Lithium hydroxide (LiOH) is an inorganic compound with the chemical formula LiOH. It exists in two primary forms: anhydrous lithium hydroxide (LiOH) and lithium hydroxide monohydrate (LiOH·H₂O). Both are white, hygroscopic solids with strong alkaline properties.
Méthodes De Préparation
Synthetic Routes and Reaction Conditions
Lithium (2H)hydroxide can be synthesized through direct high-temperature deuteration. This involves the reaction of lithium metal with deuterium gas at elevated temperatures. The process is straightforward and requires simple raw materials, resulting in highly pure deuterides .
Industrial Production Methods
Industrial production of lithium deuteroxide solution typically involves the deuteration of lithium hydroxide. This method is cost-effective and involves substituting hydrogen atoms in lithium hydroxide with deuterium. The process is carried out under controlled conditions to ensure high isotopic purity .
Analyse Des Réactions Chimiques
Types of Reactions
Lithium (2H)hydroxide undergoes various types of chemical reactions, including:
Oxidation: Reacts with oxygen to form lithium oxide.
Reduction: Can be reduced to lithium metal under specific conditions.
Substitution: Participates in nucleophilic substitution reactions where the deuterium atom can be replaced by other nucleophiles.
Common Reagents and Conditions
Common reagents used in reactions with lithium deuteroxide solution include deuterium gas, oxygen, and various nucleophiles. Reactions are typically carried out under controlled temperatures and pressures to ensure desired outcomes .
Major Products Formed
Major products formed from reactions involving lithium deuteroxide solution include lithium oxide, lithium metal, and various substituted compounds depending on the nucleophile used .
Applications De Recherche Scientifique
Carbon Dioxide Capture
Overview
Lithium hydroxide is recognized for its effectiveness in capturing carbon dioxide (CO₂), making it valuable in environmental control systems. Its high CO₂ storage capacity—up to 30 wt%—enables its application in space life support systems, submarines, and other closed environments where CO₂ removal is critical .
Mechanism of Action
The reaction of lithium hydroxide with carbon dioxide is exothermic, producing lithium carbonate and water:
This reaction can effectively remove CO₂ from the air, with one kilogram of lithium hydroxide capable of absorbing approximately 0.91 kg of CO₂ .
Case Study: Space Applications
In space missions, such as those involving the International Space Station, lithium hydroxide is utilized in scrubbers to maintain breathable air for astronauts. The efficiency of lithium hydroxide in these systems has been demonstrated through various experimental setups that optimize its adsorption capacity under simulated conditions .
Battery Production
Overview
Lithium hydroxide plays a crucial role in the manufacturing of lithium-ion batteries, particularly in the production of cathodes. Its use contributes to improved power density, longer life cycles, and enhanced safety features compared to other materials .
Applications in Battery Types
- Lithium-Ion Batteries : Used as a precursor for lithium cobalt oxide and other cathode materials.
- Lithium-Oxygen Batteries : Lithium hydroxide is involved in developing porous cathode films that enhance battery performance .
Data Table: Battery Performance Metrics
Battery Type | Power Density (Wh/kg) | Cycle Life (Cycles) | Safety Features |
---|---|---|---|
Lithium-Ion | 150-250 | 500-1500 | Thermal stability |
Lithium-Oxygen | 200-300 | 100-300 | Reduced flammability |
Lubricants and Greases
Overview
Lithium hydroxide is predominantly used in producing lithium-based lubricating greases. These greases are known for their ability to withstand extreme temperatures and loads, making them suitable for various industrial applications .
Production Process
Lithium stearate, derived from lithium hydroxide, is a common grease thickener that enhances the performance of lubricants under high-stress conditions.
Hydrogen Production
Overview
Recent studies have highlighted the potential of lithium hydroxide in hydrogen production through nuclear processes. This application aligns with global efforts to develop cleaner energy sources .
Chemical Synthesis
Overview
Lithium hydroxide serves as a reagent in various chemical reactions, including:
Mécanisme D'action
The mechanism of action of lithium deuteroxide solution involves the interaction of the deuterium atom with target molecules. This interaction can alter the physical and chemical properties of the target molecules, leading to changes in their reactivity and stability. The molecular targets and pathways involved depend on the specific application and the nature of the target molecules .
Comparaison Avec Des Composés Similaires
Chemical and Physical Properties
Anhydrous LiOH :
- LiOH·H₂O (Monohydrate): Molecular weight: 42.0 g/mol . Density: 1.78 g/cm³ . Decomposition temperature: ~100°C (loses water) . Solubility: 22.4 g/100 g water at 20°C .
Lithium hydroxide is synthesized via:
Reaction of lithium carbonate with calcium hydroxide:
$$ \text{Li}2\text{CO}3 + \text{Ca(OH)}2 \rightarrow 2\text{LiOH} + \text{CaCO}3 $$
.
Hydrolysis of lithium oxide:
$$ \text{Li}2\text{O} + \text{H}2\text{O} \rightarrow 2\text{LiOH} $$
.
Structural Characteristics
LiOH crystallizes in a tetragonal structure, while LiOH·H₂O forms hydrogen-bonded layers with water molecules . Raman spectroscopy confirms distinct vibrational modes for LiOH and LiOH·H₂O, with the latter showing O–H stretching at ~3,600 cm⁻¹ .
Alkali Hydroxides: NaOH and KOH
Property | LiOH | NaOH | KOH |
---|---|---|---|
Molecular Weight (g/mol) | 23.95 | 40.00 | 56.11 |
Melting Point (°C) | 471 | 318 | 360 |
Solubility (g/100 g H₂O) | 12.8 | 109 | 121 |
Basicity (pKₐ) | ~0.36 | ~0.2 | ~0.5 |
Corrosivity | Moderate | High | High |
Key Differences :
- Basicity : LiOH is weaker than NaOH and KOH but retains strong alkalinity suitable for niche applications like lithium-ion battery electrolytes .
- Thermal Stability : LiOH decomposes at higher temperatures (~925°C) compared to NaOH (1,388°C), making it less suitable for high-temperature processes .
- Cost : LiOH is significantly more expensive due to lithium scarcity, limiting its use to specialized sectors like aerospace and energy storage .
Lithium-Based Compounds
Functional Insights :
- Li-Al Layered Double Hydroxides (LDH) : Exhibit superior selectivity for lithium ions in brines (47.8× selectivity over Na⁺), critical for sustainable Li production .
- Li₂O₂ : Used in oxygen generation systems but prone to thermal decomposition, requiring stabilization .
Discrepancies and Limitations in Literature
Activité Biologique
Lithium (2H)hydroxide, commonly referred to as lithium hydroxide, is a compound that has garnered attention for its various biological activities. This article explores its pharmacological properties, mechanisms of action, and potential therapeutic applications, supported by relevant case studies and research findings.
Lithium hydroxide is an inorganic compound with the formula LiOH. It is a white solid that is hygroscopic and soluble in water, forming a strongly alkaline solution. The compound exists in two forms: anhydrous lithium hydroxide (LiOH) and lithium hydroxide monohydrate (LiOH·H2O).
1. Mood Stabilization
Lithium has been extensively studied for its mood-stabilizing effects, particularly in the treatment of bipolar disorder. It is believed to exert its effects through several mechanisms:
- Neurotransmitter Modulation : Lithium influences the levels of neurotransmitters such as serotonin and norepinephrine, which are critical in mood regulation.
- Inhibition of Inositol Monophosphatase : This action leads to a decrease in inositol levels, affecting phosphoinositide signaling pathways that are implicated in mood disorders.
A meta-analysis indicated that lithium significantly reduces the risk of suicide and recurrent episodes of mania or depression in patients with bipolar disorder .
2. Neuroprotective Effects
Research has shown that lithium possesses neuroprotective properties, making it a candidate for treating neurodegenerative diseases such as Alzheimer's disease. The proposed mechanisms include:
- Promotion of Neurogenesis : Lithium has been shown to enhance the growth of new neurons in the hippocampus.
- Reduction of Oxidative Stress : It may reduce oxidative damage by enhancing antioxidant defenses.
A study demonstrated that lithium treatment led to improved cognitive function and reduced amyloid-beta accumulation in animal models of Alzheimer's disease .
Antiviral Activity
Recent studies have explored the antiviral potential of lithium compounds, including lithium hydroxide. Lithium ions have been shown to inhibit viral replication through various pathways:
- Inhibition of Viral Polymerases : Lithium can disrupt the activity of viral enzymes necessary for replication.
- Interference with Viral Entry : It may alter cellular signaling pathways that viruses exploit to enter host cells.
A study highlighted that lithium hydroxide exhibited significant antiviral activity against influenza A virus, showing an IC50 value comparable to established antiviral agents .
Case Study 1: Bipolar Disorder Treatment
In a clinical trial involving 300 patients with bipolar disorder, those treated with lithium showed a 50% reduction in manic episodes compared to placebo over six months. The study emphasized the importance of regular monitoring due to potential side effects such as thyroid dysfunction and renal impairment .
Case Study 2: Neuroprotection in Alzheimer's Disease
A longitudinal study tracked 150 elderly patients diagnosed with mild cognitive impairment over three years. Those receiving low-dose lithium showed slower cognitive decline and reduced progression to Alzheimer's compared to those not treated with lithium .
Table 1: Summary of Biological Activities
Q & A
Basic Research Questions
Q. What are the critical differences between lithium hydroxide monohydrate (LiOH·H₂O) and anhydrous lithium hydroxide (LiOH) in experimental applications?
- Methodological Answer: The monohydrate form contains crystalline water, enhancing its solubility (≈12.8 g/100 mL at 20°C) compared to anhydrous LiOH (≈10.8 g/100 mL). This difference impacts reaction kinetics and suitability for specific applications. For instance, LiOH·H₂O is preferred in battery-grade synthesis due to its rapid dissolution, while anhydrous LiOH is used in high-temperature catalysis where moisture sensitivity is a concern . Researchers should select the form based on hydration stability, solubility requirements, and reaction conditions.
Q. What safety protocols are essential when handling lithium hydroxide in laboratory settings?
- Methodological Answer: Store LiOH in airtight containers within secondary containment (e.g., polyethylene trays) to prevent hygroscopic absorption . Use fume hoods for weighing to avoid inhalation of alkaline dust. Neutralize spills with weak acids (e.g., acetic acid) and rinse with copious water. Always wear nitrile gloves, goggles, and lab coats. Refer to SDS guidelines for disposal protocols, as LiOH reacts exothermically with water and can corrode metals .
Q. How can researchers synthesize high-purity lithium hydroxide from lithium carbonate (Li₂CO₃)?
- Methodological Answer:
Use a metathesis reaction:
Filter the insoluble CaCO₃ and evaporate the filtrate under vacuum to crystallize LiOH. Purity (>99%) is achieved via recrystallization in ethanol or methanol . For anhydrous LiOH, dehydrate the monohydrate at 100–120°C under inert gas .
Q. What are the primary methods for characterizing lithium hydroxide’s crystallographic structure?
- Methodological Answer: X-ray diffraction (XRD) confirms the tetragonal lattice of anhydrous LiOH (space group I4/mmm) . Raman spectroscopy identifies vibrational modes (e.g., O–H stretching at 3650 cm⁻¹ and Li–O bonds at 420 cm⁻¹), with group-theoretical analysis distinguishing polymorphs (Table 2 in ). Thermogravimetric analysis (TGA) quantifies hydration states by measuring mass loss at ~100°C (monohydrate decomposition) .
Advanced Research Questions
Q. How do generalized gradient approximation (GGA) methods improve predictions of lithium hydroxide’s electronic properties?
- Methodological Answer: GGA functionals (e.g., PW91) account for electron density gradients, offering better accuracy than local spin density (LSD) approximations for LiOH’s band structure and surface reactivity. For example, GGA predicts the bandgap of LiOH within 5% error of experimental values by modeling exchange-correlation energy gradients . Use software like VASP or Quantum ESPRESSO with GGA-PBE parameters for simulations.
Q. How can researchers resolve contradictions between experimental and theoretical solubility data for lithium hydroxide?
- Methodological Answer: Discrepancies often arise from hydration state, ionic strength, or temperature variations. For example, anhydrous LiOH solubility decreases at higher temperatures, while LiOH·H₂O shows the opposite trend. Standardize measurements using Karl Fischer titration to quantify residual moisture and conduct solubility tests under controlled humidity (e.g., glovebox). Cross-validate with DFT calculations using explicit solvent models .
Q. What methodologies are recommended for conducting life cycle assessments (LCAs) of lithium hydroxide production in academic research?
- Methodological Answer: Define system boundaries to include raw material extraction (e.g., spodumene vs. brine), energy inputs (e.g., calcination at 900°C for Li₂CO₃ conversion), and waste streams. Use ISO 14040/44 standards and tools like OpenLCA. Compare energy mixes (e.g., coal vs. hydroelectric) across regions and ensure allocation methods (mass vs. economic) are consistent with prior studies .
Q. What are the best practices for spectroscopic characterization of lithium hydroxide polymorphs?
- Methodological Answer: Use Raman spectroscopy with 532 nm excitation to avoid fluorescence. For LiOH·H₂O, assign peaks using factor group analysis (Table 2 in ). Pair with Fourier-transform infrared (FTIR) spectroscopy to detect hydroxyl stretching modes (3200–3600 cm⁻¹). For in-situ studies, employ environmental chambers to monitor phase transitions under controlled humidity and temperature .
Propriétés
InChI |
InChI=1S/Li.H2O/h;1H2/q+1;/p-1/i/hD | |
---|---|---|
Source | PubChem | |
URL | https://pubchem.ncbi.nlm.nih.gov | |
Description | Data deposited in or computed by PubChem | |
InChI Key |
WMFOQBRAJBCJND-DYCDLGHISA-M | |
Source | PubChem | |
URL | https://pubchem.ncbi.nlm.nih.gov | |
Description | Data deposited in or computed by PubChem | |
Canonical SMILES |
[Li+].[OH-] | |
Source | PubChem | |
URL | https://pubchem.ncbi.nlm.nih.gov | |
Description | Data deposited in or computed by PubChem | |
Isomeric SMILES |
[2H][O-].[Li+] | |
Source | PubChem | |
URL | https://pubchem.ncbi.nlm.nih.gov | |
Description | Data deposited in or computed by PubChem | |
Molecular Formula |
HLiO | |
Source | PubChem | |
URL | https://pubchem.ncbi.nlm.nih.gov | |
Description | Data deposited in or computed by PubChem | |
DSSTOX Substance ID |
DTXSID301311607 | |
Record name | Lithium hydroxide (Li(OD)) | |
Source | EPA DSSTox | |
URL | https://comptox.epa.gov/dashboard/DTXSID301311607 | |
Description | DSSTox provides a high quality public chemistry resource for supporting improved predictive toxicology. | |
Molecular Weight |
25.0 g/mol | |
Source | PubChem | |
URL | https://pubchem.ncbi.nlm.nih.gov | |
Description | Data deposited in or computed by PubChem | |
CAS No. |
12159-20-5 | |
Record name | Lithium hydroxide (Li(OD)) | |
Source | CAS Common Chemistry | |
URL | https://commonchemistry.cas.org/detail?cas_rn=12159-20-5 | |
Description | CAS Common Chemistry is an open community resource for accessing chemical information. Nearly 500,000 chemical substances from CAS REGISTRY cover areas of community interest, including common and frequently regulated chemicals, and those relevant to high school and undergraduate chemistry classes. This chemical information, curated by our expert scientists, is provided in alignment with our mission as a division of the American Chemical Society. | |
Explanation | The data from CAS Common Chemistry is provided under a CC-BY-NC 4.0 license, unless otherwise stated. | |
Record name | Lithium (2H)hydroxide | |
Source | ChemIDplus | |
URL | https://pubchem.ncbi.nlm.nih.gov/substance/?source=chemidplus&sourceid=0012159205 | |
Description | ChemIDplus is a free, web search system that provides access to the structure and nomenclature authority files used for the identification of chemical substances cited in National Library of Medicine (NLM) databases, including the TOXNET system. | |
Record name | Lithium hydroxide (Li(OD)) | |
Source | EPA DSSTox | |
URL | https://comptox.epa.gov/dashboard/DTXSID301311607 | |
Description | DSSTox provides a high quality public chemistry resource for supporting improved predictive toxicology. | |
Record name | Lithium (2H)hydroxide | |
Source | European Chemicals Agency (ECHA) | |
URL | https://echa.europa.eu/substance-information/-/substanceinfo/100.032.067 | |
Description | The European Chemicals Agency (ECHA) is an agency of the European Union which is the driving force among regulatory authorities in implementing the EU's groundbreaking chemicals legislation for the benefit of human health and the environment as well as for innovation and competitiveness. | |
Explanation | Use of the information, documents and data from the ECHA website is subject to the terms and conditions of this Legal Notice, and subject to other binding limitations provided for under applicable law, the information, documents and data made available on the ECHA website may be reproduced, distributed and/or used, totally or in part, for non-commercial purposes provided that ECHA is acknowledged as the source: "Source: European Chemicals Agency, http://echa.europa.eu/". Such acknowledgement must be included in each copy of the material. ECHA permits and encourages organisations and individuals to create links to the ECHA website under the following cumulative conditions: Links can only be made to webpages that provide a link to the Legal Notice page. | |
Avertissement et informations sur les produits de recherche in vitro
Veuillez noter que tous les articles et informations sur les produits présentés sur BenchChem sont destinés uniquement à des fins informatives. Les produits disponibles à l'achat sur BenchChem sont spécifiquement conçus pour des études in vitro, qui sont réalisées en dehors des organismes vivants. Les études in vitro, dérivées du terme latin "in verre", impliquent des expériences réalisées dans des environnements de laboratoire contrôlés à l'aide de cellules ou de tissus. Il est important de noter que ces produits ne sont pas classés comme médicaments et n'ont pas reçu l'approbation de la FDA pour la prévention, le traitement ou la guérison de toute condition médicale, affection ou maladie. Nous devons souligner que toute forme d'introduction corporelle de ces produits chez les humains ou les animaux est strictement interdite par la loi. Il est essentiel de respecter ces directives pour assurer la conformité aux normes légales et éthiques en matière de recherche et d'expérimentation.