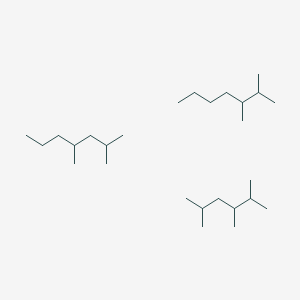
Tripropylene
Vue d'ensemble
Description
Tripropylene glycol (CASRN 24800-44-0) is an oligomeric glycol derived from the condensation of propylene oxide, containing three propylene oxide units. It is a colorless, viscous liquid with a molecular weight of 192.3 g/mol, a density of 1.110 g/cm³, and a boiling point of 265.1°C . Its primary applications span cosmetics, solvents, hydraulic fluids, flame retardants, and industrial processes such as nanoparticle synthesis and diesel additive formulations . This compound glycol is classified as low concern for acute aquatic toxicity (LC50 >100 ppm) and exhibits moderate biodegradability (half-life <60 days) .
Méthodes De Préparation
Acid-Catalyzed Oligomerization of Propylene
The most established method for tripropylene synthesis involves the acid-catalyzed oligomerization of propylene (C₃H₆). This process leverages Brønsted or Lewis acids to initiate carbocation formation, enabling sequential propylene addition. Polyphosphoric acid (PPA) is a widely used catalyst due to its ability to stabilize intermediate carbocations while minimizing side reactions .
Reaction Mechanism and Conditions
The reaction begins with protonation of propylene by the acid catalyst, generating a secondary carbocation ((CH₃)₂CH⁺). This carbocation attacks another propylene molecule, forming a larger carbocation that undergoes further propagation. Termination occurs via deprotonation or hydride transfer, yielding a mixture of nonene isomers. Typical conditions involve temperatures of 50–120°C and atmospheric pressure, though higher pressures (up to 2 MPa) may enhance propylene solubility in liquid-phase systems .
A study utilizing polyphosphoric acid at 80°C achieved a this compound selectivity of 58.6% alongside minor pentapropylene glycol byproducts (0.35%) . However, product distribution remains highly dependent on catalyst loading and reaction time. For instance, increasing the catalyst-to-propylene ratio from 1:50 to 1:20 improved this compound yield by 12% but also elevated oligomer chain lengths .
Templated Synthesis for Length-Controlled Oligomerization
Recent breakthroughs in supramolecular chemistry have enabled precise control over oligomer chain lengths. Macrocyclic templates, such as rigid oligoproline structures, align monomers spatially to dictate polymerization degrees. This approach mimics biological systems like DNA replication, where templates ensure sequence fidelity .
Catalytic Macrocyclic Templates
In a landmark study, a macrocyclic template decorated with catalytic sites facilitated the oligomerization of propylene derivatives with unprecedented specificity . The template’s cavity size predetermined the number of monomer units incorporated, yielding this compound as the dominant product (75% selectivity) at 25°C. This method eliminated the need for stoichiometric templates, operating instead with catalytic amounts (5 mol% template loading) .
Table 1: Performance Comparison of Templated vs. Acid-Catalyzed Oligomerization
Parameter | Acid-Catalyzed Method | Templated Method |
---|---|---|
Temperature (°C) | 80–120 | 25–40 |
Selectivity for C₉H₁₈ | 48–58% | 75% |
Byproduct Formation | Up to 10% | <5% |
Catalyst Loading | 10–20 wt% | 5 mol% |
Microreactor Technology for Enhanced Selectivity
Continuous-flow microreactors have emerged as a promising platform for this compound synthesis, offering superior heat and mass transfer compared to batch reactors. A patented method employing a "heart-shaped" microchannel reactor demonstrated 90% selectivity for hexafluoropropylene trimer—a fluorinated analog of this compound—at 100°C . While tailored for fluorinated monomers, this technology highlights the potential for adapting microreactors to propylene systems.
Advantages of Microreactor Systems
-
Precision Temperature Control : Microreactors maintain isothermal conditions (±2°C), minimizing thermal degradation .
-
Reduced Residence Time : Reactions complete in minutes rather than hours, curtailing side reactions.
-
Scalability : Modular designs allow parallelization for industrial-scale production .
Industrial-Scale Production and Process Optimization
Large-scale this compound manufacture typically employs continuous stirred-tank reactors (CSTRs) or tubular reactors. The hydrolysis of propylene oxide (PO) under high pressure (up to 2,170 kPa) and temperature (120–190°C) remains a cornerstone of glycol production, though this compound emerges as a byproduct in these systems . Recent patent literature describes a two-stage process where propylene glycol undergoes sequential PO addition in the presence of barium hydroxide, achieving this compound yields of 48.5–58.6% .
Table 2: Industrial Process Parameters for this compound Synthesis
Parameter | Stage 1 (Glycol Formation) | Stage 2 (Oligomerization) |
---|---|---|
Temperature (°C) | 120–190 | 85–105 |
Pressure (kPa) | 2,170 | 100–450 |
Catalyst | Water | Ba(OH)₂·H₂O |
Yield (C₉H₁₈) | N/A | 48.5–58.6% |
Analyse Des Réactions Chimiques
Hydrolysis to Glycols
Tripropylene glycol (TPG), a derivative, forms via propylene oxide (PO) hydration :
Reaction :
\text{PO}+\text{H}_2\text{O}\rightarrow \text{TPG}\(\text{this compound glycol})
Experimental Data :
Catalyst | PO Conversion (%) | TPG Selectivity (%) | Byproduct (Tetra-PG, %) |
---|---|---|---|
Niobic acid | 94 | 82 | <0.1 |
Tantalum oxide | 87 | 79 | 0.5 |
H-ZSM-5 zeolite | 68 | 26 | 3 |
Source: Sumitomo Chemical (2014)
-
Temperature Effect : At 200°C, TPG selectivity remains >80% despite increased reaction rates .
-
Molar Ratio : PO/H₂O = 2:1 maximizes TPG yield (Fig. 6 in ).
Thermal Decomposition and Combustion
This compound undergoes pyrolysis and combustion, following radical-chain mechanisms :
Primary Pathways :
-
C–C Bond Cleavage : Forms allyl radicals () and smaller alkenes.
-
H-Abstraction : Generates resonance-stabilized allylic radicals.
Kinetic Parameters :
Reaction | Rate Constant (cm³/mol·s) | Activation Energy (kJ/mol) |
---|---|---|
436.2 | ||
81.7 |
Source: NIST Chemical Kinetics Database (1991)
Alkylation Reactions
This compound serves as an alkylating agent in surfactant synthesis :
Example :
-
Solvent Effects : Polar solvents (e.g., this compound glycol) improve selectivity for linear alkylates by 30% .
-
Byproduct Control : Acidic ion-exchange resins reduce dimerization by 40% compared to AlCl₃ .
Catalytic Oxidation
Partial oxidation yields epoxides and ketones:
Reaction :
Applications De Recherche Scientifique
Industrial Applications
1.1 Polymer Production
Tripropylene glycol serves as a key raw material for producing various polymers, particularly polyesters and alkyd resins. These polymers are essential in manufacturing coatings, adhesives, and sealants.
- Polyester Resins: TPG is utilized in unsaturated polyester resins, which are widely used in the automotive and construction industries due to their excellent mechanical properties and resistance to environmental factors.
- Alkyd Resins: In the production of alkyd resins, TPG enhances the flexibility and durability of the final product, making it suitable for paints and varnishes.
1.2 Plasticizers
TPG functions as a plasticizer in polyvinyl chloride (PVC) and other polymer systems. Its low volatility and high boiling point contribute to improved processing characteristics and performance of the final products.
1.3 Solvent Applications
Due to its broad solvency power, TPG is employed as a solvent in various formulations:
- Textile Soaps and Lubricants: It is used in textile processing to enhance the quality of fabrics.
- Cutting Oils: TPG is incorporated into cutting oil formulations to improve lubrication during machining processes.
- Ink Removal Creams: Its solvency properties make it effective in formulations designed for ink removal.
Consumer Products
2.1 Cosmetics and Personal Care
this compound glycol is widely used in cosmetic formulations due to its safety profile and moisturizing properties. It acts as a humectant, helping to retain moisture in skin care products.
- Moisturizers: TPG enhances the texture and efficacy of moisturizing creams.
- Fragrances: It serves as a carrier for fragrances, improving their stability and delivery.
2.2 Household Products
In household cleaning products, TPG contributes to the formulation of effective cleaning agents due to its solvency properties.
Case Studies
3.1 Photopolymerization Processes
Recent advancements have highlighted the use of this compound glycol diacrylate (TPGDA) in photopolymerization processes for additive manufacturing. TPGDA is utilized as a reaction dilution agent in UV-cured resins, which are essential for producing complex 3D objects with high precision . This application underscores TPG's role in innovative manufacturing technologies.
3.2 Environmental Considerations
The demand for this compound glycol has increased due to its environmentally friendly profile compared to traditional solvents that emit volatile organic compounds (VOCs). Manufacturers are increasingly adopting TPG-based formulations to comply with stricter environmental regulations .
Data Table: Properties and Applications of this compound Glycol
Application Area | Specific Use | Key Benefits |
---|---|---|
Polymer Production | Polyester & Alkyd Resins | Durability & Flexibility |
Plasticizers | PVC & Other Polymers | Enhanced Processing Characteristics |
Solvent | Textile Soaps & Lubricants | Broad Solvency Power |
Cosmetics | Moisturizers & Fragrances | Moisturizing Properties |
Cleaning Agents | Household Cleaners | Effective Cleaning |
Mécanisme D'action
The mechanism of action of tripropylene involves its ability to form carbocations, which can then participate in various chemical reactions. The formation of carbocations allows this compound to act as an alkylating agent, facilitating the transfer of alkyl groups to other molecules . This property is particularly useful in the synthesis of surfactants and lubricants, where this compound can modify the chemical structure of target molecules to enhance their properties.
Comparaison Avec Des Composés Similaires
Structural and Physical-Chemical Properties
Tripropylene glycol is compared to propylene glycol (PG), dipropylene glycol (DPG), and triethylene glycol (TEG) based on structural and physical-chemical properties (Table 1).
Table 1: Physical-Chemical Comparison of this compound Glycol with Analogues
Key differences include:
- Molecular Weight and Boiling Point : this compound glycol’s higher molecular weight and boiling point compared to DPG and PG enhance its thermal stability, making it suitable for high-temperature applications .
- Solvent Performance : this compound glycol exhibits superior solvency for aromatic hydrocarbons (e.g., toluene, xylene) compared to DPG, as evidenced by vapor-liquid equilibrium (VLE) data .
Environmental Impact
- Aquatic Toxicity : this compound glycol (LC50 >100 ppm) is less toxic than PG (LC50 ~10,000 ppm) but similar to DPG .
- Biodegradability : this compound glycol degrades faster (half-life <60 days) than PG (half-life >60 days) .
Key Research Findings
- Toxicity Analogues : The U.S. EPA classifies this compound glycol and DPG as structurally analogous, with nearly identical environmental persistence and toxicity profiles .
- Performance in Polymers : this compound glycol-modified polypropylene composites exhibit enhanced thermal stability compared to DPG-based variants .
- Diesel Additives : this compound glycol methyl ether (TGME) reduces particulate emissions by 30% in diesel engines, outperforming dibutyl maleate (DBM) .
Q & A
Basic Research Questions
Q. What are the key physicochemical properties of tripropylene glycol critical for experimental design in environmental fate studies?
Methodological Answer: Key properties include molecular weight (192 g/mol), melting point (-19.99°C), and biodegradability (>60% within 28 days under aerobic conditions). These parameters inform solubility, environmental partitioning, and persistence assessments. For example, low bioaccumulation potential (predicted via EPI Suite models) reduces priority in bioaccumulation assays . Experimental designs should prioritize measuring octanol-water partition coefficients (log Kow) and vapor pressure to predict environmental behavior .
Q. How can researchers optimize synthesis protocols for this compound glycol to achieve high purity for toxicological assays?
Methodological Answer: Purification methods such as fractional distillation or chromatography are critical. Monitor purity using gas chromatography-mass spectrometry (GC-MS) and nuclear magnetic resonance (NMR). Contaminants like residual catalysts (e.g., alkali metal hydroxides) may skew toxicity results; thus, rigorous post-synthesis washing (e.g., ion-exchange resins) is recommended .
Q. What standardized assays are recommended for evaluating this compound glycol’s acute aquatic toxicity?
Methodological Answer: Use OECD Test Guidelines 201 (algae), 202 (daphnia), and 203 (fish) for acute toxicity. ECOSAR models predict a 96-hour LC50 >100 mg/L for fish, but lab validation is necessary. Include negative controls and reference toxicants (e.g., sodium lauryl sulfate) to ensure assay reliability .
Advanced Research Questions
Q. How do structural analogs (e.g., dipropylene glycol) inform hazard extrapolation for this compound glycol in data-limited scenarios?
Methodological Answer: Analog-based read-across requires alignment in functional groups, metabolic pathways, and toxicity mechanisms. For developmental toxicity, compare this compound glycol’s low concern (EPA Tier 1 assessment) with analogs’ NOAELs (No Observed Adverse Effect Levels). Validate via in vitro assays (e.g., zebrafish embryotoxicity) to address data gaps .
Q. How can conflicting data on this compound glycol’s aquatic toxicity be resolved through integrated experimental and computational approaches?
Methodological Answer: Discrepancies often arise from variations in test species, exposure durations, or impurity profiles. Apply weight-of-evidence analysis by combining experimental data (e.g., EPA’s ECOSAR outputs) with probabilistic modeling. For instance, reconcile acute vs. chronic toxicity results using species sensitivity distributions (SSDs) .
Q. What methodological frameworks are recommended for assessing this compound glycol’s persistence and bioaccumulation potential across heterogeneous environmental compartments?
Methodological Answer: Use tiered testing:
- Tier 1 : Aerobic biodegradation (OECD 301F) and EPI Suite predictions for bioaccumulation factors (BCF).
- Tier 2 : Site-specific modeling (e.g., USEtox) to account for soil-water partitioning and photodegradation kinetics.
- Tier 3 : Mesocosm studies to validate field persistence .
Q. How should researchers design studies to address potential susceptibility of subpopulations (e.g., immunocompromised individuals) to this compound glycol exposure?
Methodological Answer: Leverage epidemiological data from occupational settings (e.g., manufacturing workers) to identify exposure biomarkers. Combine with in vitro human cell models (e.g., hepatocytes, immune cells) to assess differential responses. EPA’s low hazard designation for general populations does not preclude niche susceptibilities .
Q. Data Analysis and Interpretation
Q. What statistical methods are optimal for analyzing dose-response relationships in this compound glycol toxicity studies?
Methodological Answer: Apply nonlinear regression models (e.g., four-parameter logistic curves) for EC50 calculations. Use Bayesian inference to quantify uncertainty in low-dose extrapolation. For microbiome-related endpoints (e.g., biodegradation), apply alpha-diversity metrics (Shannon index) and PERMANOVA for community shifts .
Q. How can researchers reconcile discrepancies between this compound glycol’s experimental toxicity data and computational predictions?
Methodological Answer: Perform sensitivity analysis on model inputs (e.g., log Kow, solubility). If ECOSAR underestimates toxicity, validate with QSAR (Quantitative Structure-Activity Relationship) models incorporating steric and electronic parameters. Cross-reference with ToxCast high-throughput screening data .
Q. What strategies mitigate bias when comparing this compound glycol’s environmental impacts against legacy chemicals (e.g., ethylene glycol)?
Methodological Answer: Normalize data by molar concentration, persistence half-life, and functional use profiles. Conduct life cycle assessment (LCA) to quantify cradle-to-grave impacts. Address confounding variables (e.g., metabolite toxicity) via longitudinal monitoring .
Q. Tables for Key Data Reference
Propriétés
IUPAC Name |
2,3-dimethylheptane;2,4-dimethylheptane;2,3,5-trimethylhexane | |
---|---|---|
Source | PubChem | |
URL | https://pubchem.ncbi.nlm.nih.gov | |
Description | Data deposited in or computed by PubChem | |
InChI |
InChI=1S/3C9H20/c1-7(2)6-9(5)8(3)4;1-5-6-9(4)7-8(2)3;1-5-6-7-9(4)8(2)3/h7-9H,6H2,1-5H3;2*8-9H,5-7H2,1-4H3 | |
Source | PubChem | |
URL | https://pubchem.ncbi.nlm.nih.gov | |
Description | Data deposited in or computed by PubChem | |
InChI Key |
PCINYZCOJRSWJK-UHFFFAOYSA-N | |
Source | PubChem | |
URL | https://pubchem.ncbi.nlm.nih.gov | |
Description | Data deposited in or computed by PubChem | |
Canonical SMILES |
CCCCC(C)C(C)C.CCCC(C)CC(C)C.CC(C)CC(C)C(C)C | |
Source | PubChem | |
URL | https://pubchem.ncbi.nlm.nih.gov | |
Description | Data deposited in or computed by PubChem | |
Molecular Formula |
C27H60 | |
Source | PubChem | |
URL | https://pubchem.ncbi.nlm.nih.gov | |
Description | Data deposited in or computed by PubChem | |
Molecular Weight |
384.8 g/mol | |
Source | PubChem | |
URL | https://pubchem.ncbi.nlm.nih.gov | |
Description | Data deposited in or computed by PubChem | |
Physical Description |
Tripropylene appears as a clear colorless liquid with a sharp odor. Insoluble in water and less dense than water. Hence floats on water. May irritate skin on contact. Vapors heavier than air. Inhalation of vapors may cause irritation. Prolonged inhalation may lead to breathing difficulty. Ingestion causes abdominal discomfort, nausea and diarrhea. Used to make other chemicals and as a lubricating oil additive. | |
Record name | TRIPROPYLENE | |
Source | CAMEO Chemicals | |
URL | https://cameochemicals.noaa.gov/chemical/4730 | |
Description | CAMEO Chemicals is a chemical database designed for people who are involved in hazardous material incident response and planning. CAMEO Chemicals contains a library with thousands of datasheets containing response-related information and recommendations for hazardous materials that are commonly transported, used, or stored in the United States. CAMEO Chemicals was developed by the National Oceanic and Atmospheric Administration's Office of Response and Restoration in partnership with the Environmental Protection Agency's Office of Emergency Management. | |
Explanation | CAMEO Chemicals and all other CAMEO products are available at no charge to those organizations and individuals (recipients) responsible for the safe handling of chemicals. However, some of the chemical data itself is subject to the copyright restrictions of the companies or organizations that provided the data. | |
Boiling Point |
271.94 to 287.06 °F at 760 mmHg (USCG, 1999) | |
Record name | TRIPROPYLENE | |
Source | CAMEO Chemicals | |
URL | https://cameochemicals.noaa.gov/chemical/4730 | |
Description | CAMEO Chemicals is a chemical database designed for people who are involved in hazardous material incident response and planning. CAMEO Chemicals contains a library with thousands of datasheets containing response-related information and recommendations for hazardous materials that are commonly transported, used, or stored in the United States. CAMEO Chemicals was developed by the National Oceanic and Atmospheric Administration's Office of Response and Restoration in partnership with the Environmental Protection Agency's Office of Emergency Management. | |
Explanation | CAMEO Chemicals and all other CAMEO products are available at no charge to those organizations and individuals (recipients) responsible for the safe handling of chemicals. However, some of the chemical data itself is subject to the copyright restrictions of the companies or organizations that provided the data. | |
Flash Point |
75 °F (USCG, 1999) | |
Record name | TRIPROPYLENE | |
Source | CAMEO Chemicals | |
URL | https://cameochemicals.noaa.gov/chemical/4730 | |
Description | CAMEO Chemicals is a chemical database designed for people who are involved in hazardous material incident response and planning. CAMEO Chemicals contains a library with thousands of datasheets containing response-related information and recommendations for hazardous materials that are commonly transported, used, or stored in the United States. CAMEO Chemicals was developed by the National Oceanic and Atmospheric Administration's Office of Response and Restoration in partnership with the Environmental Protection Agency's Office of Emergency Management. | |
Explanation | CAMEO Chemicals and all other CAMEO products are available at no charge to those organizations and individuals (recipients) responsible for the safe handling of chemicals. However, some of the chemical data itself is subject to the copyright restrictions of the companies or organizations that provided the data. | |
CAS No. |
13987-01-4 | |
Record name | TRIPROPYLENE | |
Source | CAMEO Chemicals | |
URL | https://cameochemicals.noaa.gov/chemical/4730 | |
Description | CAMEO Chemicals is a chemical database designed for people who are involved in hazardous material incident response and planning. CAMEO Chemicals contains a library with thousands of datasheets containing response-related information and recommendations for hazardous materials that are commonly transported, used, or stored in the United States. CAMEO Chemicals was developed by the National Oceanic and Atmospheric Administration's Office of Response and Restoration in partnership with the Environmental Protection Agency's Office of Emergency Management. | |
Explanation | CAMEO Chemicals and all other CAMEO products are available at no charge to those organizations and individuals (recipients) responsible for the safe handling of chemicals. However, some of the chemical data itself is subject to the copyright restrictions of the companies or organizations that provided the data. | |
Record name | 1-Propene, trimer | |
Source | ChemIDplus | |
URL | https://pubchem.ncbi.nlm.nih.gov/substance/?source=chemidplus&sourceid=0013987014 | |
Description | ChemIDplus is a free, web search system that provides access to the structure and nomenclature authority files used for the identification of chemical substances cited in National Library of Medicine (NLM) databases, including the TOXNET system. | |
Retrosynthesis Analysis
AI-Powered Synthesis Planning: Our tool employs the Template_relevance Pistachio, Template_relevance Bkms_metabolic, Template_relevance Pistachio_ringbreaker, Template_relevance Reaxys, Template_relevance Reaxys_biocatalysis model, leveraging a vast database of chemical reactions to predict feasible synthetic routes.
One-Step Synthesis Focus: Specifically designed for one-step synthesis, it provides concise and direct routes for your target compounds, streamlining the synthesis process.
Accurate Predictions: Utilizing the extensive PISTACHIO, BKMS_METABOLIC, PISTACHIO_RINGBREAKER, REAXYS, REAXYS_BIOCATALYSIS database, our tool offers high-accuracy predictions, reflecting the latest in chemical research and data.
Strategy Settings
Precursor scoring | Relevance Heuristic |
---|---|
Min. plausibility | 0.01 |
Model | Template_relevance |
Template Set | Pistachio/Bkms_metabolic/Pistachio_ringbreaker/Reaxys/Reaxys_biocatalysis |
Top-N result to add to graph | 6 |
Feasible Synthetic Routes
Avertissement et informations sur les produits de recherche in vitro
Veuillez noter que tous les articles et informations sur les produits présentés sur BenchChem sont destinés uniquement à des fins informatives. Les produits disponibles à l'achat sur BenchChem sont spécifiquement conçus pour des études in vitro, qui sont réalisées en dehors des organismes vivants. Les études in vitro, dérivées du terme latin "in verre", impliquent des expériences réalisées dans des environnements de laboratoire contrôlés à l'aide de cellules ou de tissus. Il est important de noter que ces produits ne sont pas classés comme médicaments et n'ont pas reçu l'approbation de la FDA pour la prévention, le traitement ou la guérison de toute condition médicale, affection ou maladie. Nous devons souligner que toute forme d'introduction corporelle de ces produits chez les humains ou les animaux est strictement interdite par la loi. Il est essentiel de respecter ces directives pour assurer la conformité aux normes légales et éthiques en matière de recherche et d'expérimentation.