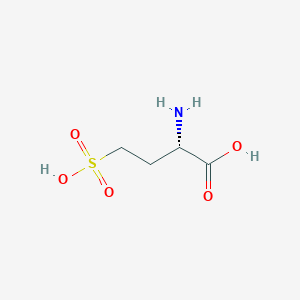
Acide L-homocystéique
Vue d'ensemble
Description
L'acide L-homocystéique est un composé organosulfuré de formule chimique HO₃SCH₂CH₂CH(NH₂)CO₂H. C'est un solide blanc ou incolore et un acide aminé non protéinogène contenant un groupe acide sulfonique. Ce composé est un analogue de l'acide glutamique et est connu pour son activité puissante en tant qu'agoniste du récepteur N-méthyl-D-aspartate (NMDA) . Il est lié à l'homocystéine, un sous-produit du métabolisme de la méthionine .
Applications De Recherche Scientifique
L-Homocysteic acid has several scientific research applications:
Neuroscience: It is used as a tool to study NMDA receptor function due to its potent agonist activity. Researchers use it to investigate the role of NMDA receptors in synaptic transmission and neurotoxicity.
Pathological Studies: The compound is employed in studies related to neurological disorders, such as epilepsy and neurodegenerative diseases.
Biochemistry: L-Homocysteic acid is used to study the metabolism of sulfur-containing amino acids and their role in cellular processes.
Mécanisme D'action
Target of Action
L-Homocysteic acid (L-HCA) is an endogenous excitatory amino acid that primarily targets the NMDA (N-methyl-D-aspartate) receptors . These receptors are a type of ionotropic glutamate receptor and play a crucial role in controlling synaptic plasticity and memory function.
Mode of Action
L-HCA acts as an agonist for the NMDA receptors . It binds to these receptors and activates them, leading to an influx of calcium ions into the cell . L-HCA is also an analog of glutamate and can competitively suppress the glutamate-cystine antiporter function in neurons, causing a decrease in cysteine uptake .
Biochemical Pathways
L-HCA is synthesized from methionine through a series of biochemical reactions involving S-adenosyl-L-methionine (SAM)-dependent transmethylation, folate-dependent or -independent remethylation cycles, and transsulfuration reactions . These pathways are crucial for regulating methionine availability, protein homeostasis, and DNA methylation .
Pharmacokinetics
It is known that l-hca can be administered intraperitoneally .
Result of Action
The activation of NMDA receptors by L-HCA can lead to various cellular effects. For instance, it can induce large currents in Purkinje cells and potently induce an acute excitotoxic reaction in ex vivo chick embryo retina . In rat primary neurons, L-HCA can induce a concentration-dependent neurotoxic effect .
Analyse Biochimique
Biochemical Properties
L-Homocysteic acid is an endogenous neurotransmitter ligand of NMDA (N-methyl-D-aspartate) receptors . It is an analog of glutamate and competitively suppresses glutamate-cystine (xc-) antiporter function in neurons, causing a decrease in cysteine uptake . It activates the NMDA receptor with an EC50 value of 14 μM .
Cellular Effects
L-Homocysteic acid has been found to increase the intracellular level of free radicals when incubated with cerebellar neurons at a concentration of 500 μM for 30 min . It also impairs glutamate uptake, redox/mitochondrial homeostasis, inflammatory response, and cell signaling pathways .
Molecular Mechanism
L-Homocysteic acid acts as a potent NMDA receptor agonist . It is an analog of glutamate and competitively suppresses glutamate-cystine (xc-) antiporter function in neurons, causing a decrease in cysteine uptake . This suggests that L-Homocysteic acid can interact with these receptors and influence their function.
Temporal Effects in Laboratory Settings
Incubation of cerebellar neurons with L-Homocysteic acid at a concentration of 500 μM for 30 min increases the intracellular level of free radicals . This suggests that the effects of L-Homocysteic acid can change over time in laboratory settings.
Metabolic Pathways
L-Homocysteic acid is related to homocysteine, a by-product of methionine metabolism . It is a part of the methionine cycle, which is crucial for regulating methionine availability, protein homeostasis, and DNA methylation .
Transport and Distribution
Given its role as an endogenous neurotransmitter ligand of NMDA receptors , it is likely that it is transported and distributed in a manner similar to other neurotransmitters.
Subcellular Localization
The subcellular localization of L-Homocysteic acid is not explicitly documented in the literature. As an endogenous neurotransmitter ligand of NMDA receptors , it is likely to be localized in the synaptic cleft where it can interact with these receptors.
Méthodes De Préparation
L'acide L-homocystéique est généralement préparé par l'oxydation de l'homocystine avec du brome aqueux . La réaction implique la conversion de l'homocystine, un dimère de l'homocystéine, en this compound par l'introduction d'un groupe acide sulfonique. Cette méthode est couramment utilisée en laboratoire pour la synthèse de l'this compound.
Analyse Des Réactions Chimiques
L'acide L-homocystéique subit diverses réactions chimiques, notamment :
Oxydation : Le composé peut être davantage oxydé pour produire des dérivés d'acide sulfonique.
Réduction : Les réactions de réduction peuvent convertir l'this compound en homocystéine ou en d'autres composés apparentés.
Substitution : Les groupes amino et carboxyle de l'this compound peuvent participer à des réactions de substitution, formant divers dérivés.
Les réactifs couramment utilisés dans ces réactions comprennent le brome pour l'oxydation et des agents réducteurs comme l'hydrogène gazeux ou les hydrures métalliques pour la réduction. Les principaux produits formés à partir de ces réactions dépendent des conditions spécifiques et des réactifs utilisés.
4. Applications de recherche scientifique
L'this compound a plusieurs applications de recherche scientifique :
Neurosciences : Il est utilisé comme outil pour étudier la fonction du récepteur NMDA en raison de son activité agoniste puissante. Les chercheurs l'utilisent pour étudier le rôle des récepteurs NMDA dans la transmission synaptique et la neurotoxicité.
Études pathologiques : Le composé est utilisé dans des études relatives aux troubles neurologiques, tels que l'épilepsie et les maladies neurodégénératives.
5. Mécanisme d'action
L'this compound exerce ses effets principalement par son action en tant qu'agoniste du récepteur NMDA . Lorsqu'il se lie aux récepteurs NMDA, il déclenche une neurotransmission excitatrice en permettant l'afflux d'ions calcium dans les neurones. Ce processus est crucial pour la plasticité synaptique, l'apprentissage et la mémoire. une activation excessive des récepteurs NMDA par l'this compound peut entraîner une neurotoxicité et la mort cellulaire, ce qui explique pourquoi il est également étudié dans le contexte des maladies neurodégénératives .
Comparaison Avec Des Composés Similaires
L'acide L-homocystéique est similaire à d'autres acides aminés soufrés, tels que :
L-Cystéine : Un précurseur de la synthèse du glutathion et impliqué dans les processus de détoxification.
Acide L-cystéine sulfinique : Un intermédiaire dans le métabolisme de la cystéine et de la taurine.
Acide L-cystéique : Un autre acide aminé soufré présentant des propriétés neuroexcitatoires.
Ce qui distingue l'this compound, c'est son activité puissante en tant qu'agoniste du récepteur NMDA, ce qui en fait un outil précieux dans la recherche en neurosciences .
Activité Biologique
L-Homocysteic acid (L-HCA) is a sulfur-containing amino acid analog of glutamic acid, primarily recognized for its role as an endogenous ligand at the N-methyl-D-aspartate (NMDA) receptor. This compound has garnered significant attention in neurobiology due to its excitotoxic properties and potential implications in neurodegenerative diseases such as Alzheimer's disease (AD).
L-Homocysteic acid is classified as an L-alpha-amino acid with a molecular formula of C4H9NO5S. Its chemical structure includes a sulfonic acid group, which contributes to its biological activity. The compound's IUPAC name is (2S)-2-amino-4-sulfobutanoic acid, and it has a CAS Registry Number of 14857-77-3.
Property | Value |
---|---|
Molecular Weight | 183.02 g/mol |
SMILES | NC@@HC(O)=O |
InChI Key | VBOQYPQEPHKASR-VKHMYHEASA-N |
L-HCA acts primarily as an agonist of the NMDA receptor, which is crucial for synaptic plasticity and memory function. Research indicates that L-HCA can induce neurotoxicity similar to that caused by NMDA but distinct from other excitatory amino acids like kainic acid. This neurotoxic effect is mediated through calcium-dependent mechanisms that lead to cytopathological changes in neuronal cells .
Neurotoxicity Studies
A significant study demonstrated that L-HCA induces a pattern of cytopathology in the ex vivo chick retina, mirroring NMDA-induced neurotoxicity. Furthermore, known NMDA antagonists effectively block the neurotoxic actions of L-HCA, reinforcing the hypothesis that L-HCA functions as an endogenous NMDA receptor ligand .
Alzheimer's Disease and Cognitive Impairment
Recent findings have linked elevated levels of plasma L-HCA to mild cognitive impairment (MCI) and Alzheimer's disease. A study indicated that plasma HCA concentrations were significantly higher in individuals with MCI compared to healthy controls, suggesting its potential role as an early diagnostic marker for cognitive decline . The sensitivity and specificity of plasma HCA in distinguishing MCI from normal cognition were reported at 95.7% and 70%, respectively.
In animal models, particularly the 3xTg-AD mouse model, increased levels of L-HCA were associated with cognitive deficits and neurodegenerative changes. Treatment with anti-homocysteic acid antibodies was found to mitigate these cognitive impairments and reduce amyloid pathology, suggesting that targeting L-HCA may offer therapeutic benefits in AD .
Case Studies
- Mild Cognitive Impairment Detection : A study involving plasma samples showed that L-HCA levels could effectively differentiate between MCI and normal cognition, highlighting its diagnostic potential in early-stage Alzheimer's pathology .
- Neuroprotective Effects : In a controlled trial with 3xTg-AD mice, administration of anti-homocysteic acid antibodies significantly reduced cognitive decline associated with vitamin B6 deficiency, indicating a protective role against neurodegeneration linked to elevated L-HCA levels .
Summary of Findings
The biological activity of L-homocysteic acid underscores its dual role as both a neurotransmitter and a potential neurotoxin linked to excitotoxicity via NMDA receptor activation. Its association with cognitive impairment and neurodegenerative diseases positions it as a critical target for future research aimed at understanding and potentially mitigating the effects of these conditions.
Table 1: Summary of Neurotoxic Effects
Agent | Neurotoxic Mechanism | Blocked By |
---|---|---|
L-Homocysteic Acid | NMDA receptor activation | Mg++, D-aminophosphonopentanoate |
NMDA | Calcium influx leading to cell death | Various NMDA antagonists |
Kainic Acid | Different pathway | Limited overlap with NMDA blockers |
Table 2: Diagnostic Performance of Plasma HCA
Condition | Sensitivity (%) | Specificity (%) |
---|---|---|
Mild Cognitive Impairment vs Normal Cognition | 95.7 | 70 |
Propriétés
IUPAC Name |
(2S)-2-amino-4-sulfobutanoic acid | |
---|---|---|
Source | PubChem | |
URL | https://pubchem.ncbi.nlm.nih.gov | |
Description | Data deposited in or computed by PubChem | |
InChI |
InChI=1S/C4H9NO5S/c5-3(4(6)7)1-2-11(8,9)10/h3H,1-2,5H2,(H,6,7)(H,8,9,10)/t3-/m0/s1 | |
Source | PubChem | |
URL | https://pubchem.ncbi.nlm.nih.gov | |
Description | Data deposited in or computed by PubChem | |
InChI Key |
VBOQYPQEPHKASR-VKHMYHEASA-N | |
Source | PubChem | |
URL | https://pubchem.ncbi.nlm.nih.gov | |
Description | Data deposited in or computed by PubChem | |
Canonical SMILES |
C(CS(=O)(=O)O)C(C(=O)O)N | |
Source | PubChem | |
URL | https://pubchem.ncbi.nlm.nih.gov | |
Description | Data deposited in or computed by PubChem | |
Isomeric SMILES |
C(CS(=O)(=O)O)[C@@H](C(=O)O)N | |
Source | PubChem | |
URL | https://pubchem.ncbi.nlm.nih.gov | |
Description | Data deposited in or computed by PubChem | |
Molecular Formula |
C4H9NO5S | |
Source | PubChem | |
URL | https://pubchem.ncbi.nlm.nih.gov | |
Description | Data deposited in or computed by PubChem | |
DSSTOX Substance ID |
DTXSID101020602 | |
Record name | (2S)-2-Amino-4-sulfobutanoic acid | |
Source | EPA DSSTox | |
URL | https://comptox.epa.gov/dashboard/DTXSID101020602 | |
Description | DSSTox provides a high quality public chemistry resource for supporting improved predictive toxicology. | |
Molecular Weight |
183.19 g/mol | |
Source | PubChem | |
URL | https://pubchem.ncbi.nlm.nih.gov | |
Description | Data deposited in or computed by PubChem | |
Physical Description |
Solid | |
Record name | L-Homocysteic acid | |
Source | Human Metabolome Database (HMDB) | |
URL | http://www.hmdb.ca/metabolites/HMDB0002205 | |
Description | The Human Metabolome Database (HMDB) is a freely available electronic database containing detailed information about small molecule metabolites found in the human body. | |
Explanation | HMDB is offered to the public as a freely available resource. Use and re-distribution of the data, in whole or in part, for commercial purposes requires explicit permission of the authors and explicit acknowledgment of the source material (HMDB) and the original publication (see the HMDB citing page). We ask that users who download significant portions of the database cite the HMDB paper in any resulting publications. | |
CAS No. |
14857-77-3 | |
Record name | Homocysteic acid | |
Source | CAS Common Chemistry | |
URL | https://commonchemistry.cas.org/detail?cas_rn=14857-77-3 | |
Description | CAS Common Chemistry is an open community resource for accessing chemical information. Nearly 500,000 chemical substances from CAS REGISTRY cover areas of community interest, including common and frequently regulated chemicals, and those relevant to high school and undergraduate chemistry classes. This chemical information, curated by our expert scientists, is provided in alignment with our mission as a division of the American Chemical Society. | |
Explanation | The data from CAS Common Chemistry is provided under a CC-BY-NC 4.0 license, unless otherwise stated. | |
Record name | L-Homocysteic acid | |
Source | ChemIDplus | |
URL | https://pubchem.ncbi.nlm.nih.gov/substance/?source=chemidplus&sourceid=0014857773 | |
Description | ChemIDplus is a free, web search system that provides access to the structure and nomenclature authority files used for the identification of chemical substances cited in National Library of Medicine (NLM) databases, including the TOXNET system. | |
Record name | (2S)-2-Amino-4-sulfobutanoic acid | |
Source | EPA DSSTox | |
URL | https://comptox.epa.gov/dashboard/DTXSID101020602 | |
Description | DSSTox provides a high quality public chemistry resource for supporting improved predictive toxicology. | |
Record name | L-HOMOCYSTEIC ACID | |
Source | FDA Global Substance Registration System (GSRS) | |
URL | https://gsrs.ncats.nih.gov/ginas/app/beta/substances/YW13F86W9W | |
Description | The FDA Global Substance Registration System (GSRS) enables the efficient and accurate exchange of information on what substances are in regulated products. Instead of relying on names, which vary across regulatory domains, countries, and regions, the GSRS knowledge base makes it possible for substances to be defined by standardized, scientific descriptions. | |
Explanation | Unless otherwise noted, the contents of the FDA website (www.fda.gov), both text and graphics, are not copyrighted. They are in the public domain and may be republished, reprinted and otherwise used freely by anyone without the need to obtain permission from FDA. Credit to the U.S. Food and Drug Administration as the source is appreciated but not required. | |
Record name | L-Homocysteic acid | |
Source | Human Metabolome Database (HMDB) | |
URL | http://www.hmdb.ca/metabolites/HMDB0002205 | |
Description | The Human Metabolome Database (HMDB) is a freely available electronic database containing detailed information about small molecule metabolites found in the human body. | |
Explanation | HMDB is offered to the public as a freely available resource. Use and re-distribution of the data, in whole or in part, for commercial purposes requires explicit permission of the authors and explicit acknowledgment of the source material (HMDB) and the original publication (see the HMDB citing page). We ask that users who download significant portions of the database cite the HMDB paper in any resulting publications. | |
Melting Point |
261 °C | |
Record name | L-Homocysteic acid | |
Source | Human Metabolome Database (HMDB) | |
URL | http://www.hmdb.ca/metabolites/HMDB0002205 | |
Description | The Human Metabolome Database (HMDB) is a freely available electronic database containing detailed information about small molecule metabolites found in the human body. | |
Explanation | HMDB is offered to the public as a freely available resource. Use and re-distribution of the data, in whole or in part, for commercial purposes requires explicit permission of the authors and explicit acknowledgment of the source material (HMDB) and the original publication (see the HMDB citing page). We ask that users who download significant portions of the database cite the HMDB paper in any resulting publications. | |
Retrosynthesis Analysis
AI-Powered Synthesis Planning: Our tool employs the Template_relevance Pistachio, Template_relevance Bkms_metabolic, Template_relevance Pistachio_ringbreaker, Template_relevance Reaxys, Template_relevance Reaxys_biocatalysis model, leveraging a vast database of chemical reactions to predict feasible synthetic routes.
One-Step Synthesis Focus: Specifically designed for one-step synthesis, it provides concise and direct routes for your target compounds, streamlining the synthesis process.
Accurate Predictions: Utilizing the extensive PISTACHIO, BKMS_METABOLIC, PISTACHIO_RINGBREAKER, REAXYS, REAXYS_BIOCATALYSIS database, our tool offers high-accuracy predictions, reflecting the latest in chemical research and data.
Strategy Settings
Precursor scoring | Relevance Heuristic |
---|---|
Min. plausibility | 0.01 |
Model | Template_relevance |
Template Set | Pistachio/Bkms_metabolic/Pistachio_ringbreaker/Reaxys/Reaxys_biocatalysis |
Top-N result to add to graph | 6 |
Feasible Synthetic Routes
Avertissement et informations sur les produits de recherche in vitro
Veuillez noter que tous les articles et informations sur les produits présentés sur BenchChem sont destinés uniquement à des fins informatives. Les produits disponibles à l'achat sur BenchChem sont spécifiquement conçus pour des études in vitro, qui sont réalisées en dehors des organismes vivants. Les études in vitro, dérivées du terme latin "in verre", impliquent des expériences réalisées dans des environnements de laboratoire contrôlés à l'aide de cellules ou de tissus. Il est important de noter que ces produits ne sont pas classés comme médicaments et n'ont pas reçu l'approbation de la FDA pour la prévention, le traitement ou la guérison de toute condition médicale, affection ou maladie. Nous devons souligner que toute forme d'introduction corporelle de ces produits chez les humains ou les animaux est strictement interdite par la loi. Il est essentiel de respecter ces directives pour assurer la conformité aux normes légales et éthiques en matière de recherche et d'expérimentation.