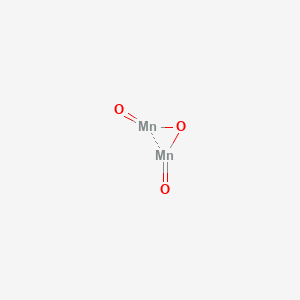
Manganese(III) oxide
- Cliquez sur DEMANDE RAPIDE pour recevoir un devis de notre équipe d'experts.
- Avec des produits de qualité à un prix COMPÉTITIF, vous pouvez vous concentrer davantage sur votre recherche.
Vue d'ensemble
Description
It occurs naturally as the mineral bixbyite and is used in various industrial applications, including the production of ferrites and thermistors . This compound is known for its brown or black crystalline appearance and has a density of 4.50 g/cm³ .
Synthetic Routes and Reaction Conditions:
Heating Manganese(IV) oxide: Manganese(III) oxide can be synthesized by heating manganese(IV) oxide (MnO₂) in air at temperatures below 800°C. This process produces α-Mn₂O₃.
Oxidation of Manganese(II) hydroxide: Another method involves the oxidation followed by dehydration of manganese(II) hydroxide (Mn(OH)₂) to produce γ-Mn₂O₃.
Redox Reactions: this compound can also be formed by the redox reaction in an alkaline cell: 2 MnO₂ + Zn → Mn₂O₃ + ZnO.
Industrial Production Methods:
In-situ Oxidation: Industrially, this compound can be prepared by the in-situ oxidation of manganese sulfate solution using oxidants such as hydrogen peroxide, sodium hypochlorite, potassium permanganate, and oxygen. This method is efficient and results in high-purity manganese oxide.
Types of Reactions:
Oxidation: this compound can undergo oxidation reactions, where it acts as an oxidizing agent.
Catalytic Reactions: this compound acts as a catalyst in various reactions, including the oxidation of methane and carbon monoxide, the decomposition of nitrogen monoxide, and the reduction of nitrobenzene.
Common Reagents and Conditions:
Oxidizing Agents: Hydrogen peroxide, sodium hypochlorite, and potassium permanganate are commonly used oxidizing agents in the preparation and reactions involving this compound.
Reducing Agents: Zinc is often used as a reducing agent in redox reactions involving this compound.
Major Products:
Applications De Recherche Scientifique
Electronics and Energy Storage
Supercapacitors and Batteries
Manganese(III) oxide is utilized in the development of supercapacitors and batteries, particularly zinc-ion batteries. Its high specific capacitance and stability make it an attractive material for energy storage devices. The compound can enhance the electrochemical performance of electrodes due to its favorable redox properties .
Application | Type | Properties |
---|---|---|
Supercapacitors | Electrodes | High specific capacitance |
Zinc-ion batteries | Anodes | Improved cycling stability |
Thermistors
this compound is also employed in thermistors, which are temperature-sensitive resistors. The material’s resistance changes significantly with temperature variations, making it suitable for temperature sensing applications .
Catalysis
This compound serves as a catalyst in various chemical reactions. It has been shown to be effective in:
- Oxidation Reactions : Mn2O3 can catalyze the oxidation of organic compounds, enhancing reaction rates and selectivity.
- Environmental Remediation : The compound is used in catalytic converters to reduce harmful emissions from vehicles by facilitating the oxidation of pollutants .
Biomedical Applications
Nanomedicine
Recent studies have highlighted the potential of manganese oxide nanomaterials (including Mn2O3) in biomedical fields such as:
- Bioimaging : Manganese oxides are used as contrast agents in MRI due to their paramagnetic properties.
- Therapeutic Applications : They are being explored for drug delivery systems and photothermal therapy for cancer treatment. Their ability to generate reactive oxygen species (ROS) makes them suitable for inducing apoptosis in cancer cells .
Case Study 1: Manganese Oxide Nanocomposites
Research has demonstrated that combining manganese oxide with biochar enhances its catalytic properties for wastewater treatment. The composite showed improved adsorption capacity for heavy metals, suggesting a dual role in catalysis and sorption .
Case Study 2: Photothermal Therapy
A study reported that manganese oxide nanoparticles could effectively convert near-infrared light into heat, leading to localized hyperthermia in tumor tissues. This approach demonstrated significant tumor regression in animal models, indicating potential for clinical applications in cancer therapy .
Mécanisme D'action
The mechanism by which manganese(III) oxide exerts its effects involves its ability to act as an oxidizing agent and catalyst. In advanced oxidation processes, this compound activates peroxymonosulfate and peroxydisulfate to generate sulfate and hydroxyl radicals, which degrade organic pollutants . The compound’s catalytic activity is attributed to its ability to facilitate electron transfer reactions, thereby accelerating the oxidation and reduction processes .
Comparaison Avec Des Composés Similaires
Manganese(III) oxide can be compared with other manganese oxides, such as:
Manganese(II) oxide (MnO): This compound is a basic oxide and is less oxidizing compared to this compound.
Manganese(II,III) oxide (Mn₃O₄): This mixed-valence oxide has both +2 and +3 oxidation states of manganese and exhibits different catalytic properties.
Manganese dioxide (MnO₂): Known for its strong oxidizing properties, manganese dioxide is widely used in batteries and as a catalyst.
This compound is unique due to its specific oxidation state (+3), which allows it to participate in a variety of redox reactions and catalytic processes. Its ability to activate peroxymonosulfate and peroxydisulfate in water treatment applications sets it apart from other manganese oxides .
Activité Biologique
Manganese(III) oxide (Mn₂O₃) and its derivatives, including manganese oxide nanoparticles (MnOₓ NPs), have garnered significant attention in recent years due to their diverse biological activities. This article explores the biological implications, enzyme-like activities, and potential applications of this compound, supported by case studies and research findings.
Overview of this compound
This compound exists in various forms, primarily as Mn₂O₃, which can exhibit different oxidation states and structural configurations. These variations influence their catalytic properties and biological interactions. The biological activity of manganese oxides is largely attributed to their ability to mimic enzyme functions, particularly in redox reactions.
Enzyme-like Activities
Research indicates that manganese oxides possess several enzyme-like activities, including:
- Oxidase-like Activity : Catalyzes the oxidation of substrates, often producing reactive oxygen species (ROS).
- Catalase-like Activity : Decomposes hydrogen peroxide (H₂O₂) into water and oxygen.
- Superoxide Dismutase (SOD)-like Activity : Converts superoxide radicals into less harmful molecules.
A study using electron spin resonance (ESR) spectroscopy demonstrated that MnOₓ NPs exhibit both pro-oxidant and anti-oxidant activities depending on their concentration and specific form. For instance, Mn₂O₃ showed the strongest ascorbate oxidation activity, while MnO₂ was more efficient in decomposing H₂O₂ .
Cytotoxicity Studies
Manganese oxides have been shown to induce cytotoxic effects in various cell lines. A comprehensive study assessed the impact of different MnOₓ NPs on three mammalian cell lines: A549 (lung cancer), HepG2 (liver cancer), and J774A.1 (macrophages). The results indicated that all tested nanoparticles exhibited concentration-dependent cytotoxicity, with notable production of ROS leading to cell death via apoptosis pathways involving caspase activation .
Cell Line | IC50 Value (μM) | Mechanism of Action |
---|---|---|
A549 | 50 | ROS production, caspase 3 activation |
HepG2 | 70 | Oxidative stress-induced apoptosis |
J774A.1 | 60 | Inflammatory response activation |
Case Studies
- Caco-2 Cell Study : In a study involving Caco-2 cells, exposure to MnOₓ NPs at concentrations below 100 μM resulted in decreased H₂O₂-induced cytotoxicity, suggesting a protective effect against oxidative stress .
- Environmental Impact : Manganese oxides are also involved in biogeochemical cycles. They can influence the availability of nutrients and metals in the environment through their redox activity, particularly in microbial processes that oxidize Mn(II) to Mn(III/IV) .
Applications in Medicine and Environmental Science
The dual nature of manganese oxides as both pro-oxidants and anti-oxidants opens avenues for their application in therapeutic contexts:
- Cancer Therapy : Due to their ROS-generating capabilities, manganese oxides can be employed in targeted cancer therapies where oxidative stress is utilized to induce apoptosis in cancer cells.
- Pollutant Degradation : Manganese(III) oxides have been shown to activate peroxymonosulfate (PMS) for the degradation of organic pollutants in wastewater treatment processes .
Propriétés
Numéro CAS |
1317-34-6 |
---|---|
Formule moléculaire |
Mn2O3 |
Poids moléculaire |
157.874 g/mol |
Nom IUPAC |
manganese(3+);oxygen(2-) |
InChI |
InChI=1S/2Mn.3O/q2*+3;3*-2 |
Clé InChI |
TYTHZVVGVFAQHF-UHFFFAOYSA-N |
SMILES |
O=[Mn]O[Mn]=O |
SMILES isomérique |
O=[Mn]O[Mn]=O |
SMILES canonique |
[O-2].[O-2].[O-2].[Mn+3].[Mn+3] |
Key on ui other cas no. |
1317-34-6 |
Pictogrammes |
Irritant |
Origine du produit |
United States |
Q1: What is the molecular formula and weight of manganese(III) oxide?
A1: The molecular formula of this compound is Mn2O3. Its molecular weight is 157.87 g/mol. [] [https://www.semanticscholar.org/paper/0b46f4176aafb500ed9607ba3e40330d4fdb3636]
Q2: What spectroscopic techniques are helpful in characterizing this compound?
A2: Several spectroscopic techniques can be used to characterize this compound, including:
- X-ray powder diffraction (XRD): This technique identifies the crystalline phases present in the sample and provides information about its crystal structure. [, , , ] [https://www.semanticscholar.org/paper/a5a1d2772c69534bf75716cbacc988b69bd0028f, https://www.semanticscholar.org/paper/db05dad3edaaddb5845a8ac21d2d018f6a8988c3, https://www.semanticscholar.org/paper/1a8b6c122ade1ebfae7c71b96db1e39c2ee4dc33, https://www.semanticscholar.org/paper/0fce317729a6ca4ab8c8eafa1cdc07442cd98179]
- Infrared spectroscopy (IR): This method identifies functional groups present in the sample, such as metal-oxygen bonds. It can also provide information about the adsorption of molecules on the surface of the oxide. [, , ] [https://www.semanticscholar.org/paper/a5a1d2772c69534bf75716cbacc988b69bd0028f, https://www.semanticscholar.org/paper/db05dad3edaaddb5845a8ac21d2d018f6a8988c3, https://www.semanticscholar.org/paper/1a8b6c122ade1ebfae7c71b96db1e39c2ee4dc33]
- Electron spectroscopy: Techniques like X-ray photoelectron spectroscopy (XPS) can determine the oxidation state of manganese in the sample and provide information about its surface composition. [] [https://www.semanticscholar.org/paper/5e6553c350f02159afdd2b2f8e3821cd817930da]
- Scanning electron microscopy (SEM): This technique provides images of the sample's surface morphology, revealing details about its shape, size, and texture. [, , , ] [https://www.semanticscholar.org/paper/5e6553c350f02159afdd2b2f8e3821cd817930da, https://www.semanticscholar.org/paper/db05dad3edaaddb5845a8ac21d2d018f6a8988c3, https://www.semanticscholar.org/paper/c67b55a23eab5c6fe2c1b2f95743f42d12bb6a53, https://www.semanticscholar.org/paper/1a8b6c122ade1ebfae7c71b96db1e39c2ee4dc33]
Q3: this compound acts as a catalyst in various reactions. Could you elaborate on some of them and their mechanisms?
A3: this compound exhibits catalytic activity in a variety of reactions, including:
- Oxidation of hydrocarbons: Studies demonstrate its ability to catalyze the oxidation of ethane by nitrous oxide and oxygen. The proposed mechanism involves the interaction of adsorbed species, likely radical species, on the catalyst surface, with both ethane and the oxidizing gas participating in subsequent steps. [, , ] [https://www.semanticscholar.org/paper/5e6553c350f02159afdd2b2f8e3821cd817930da, https://www.semanticscholar.org/paper/fbbecfada0598608758f6b628d0bb19272594a37, https://www.semanticscholar.org/paper/de25737870fc689ff837e4acdbd75e26d617e8cc]
- Oxidation of carbon monoxide: Research indicates that this compound, in conjunction with palladium, exhibits cooperative action in catalyzing the oxidation of carbon monoxide. This process involves oxygen incorporation through this compound, followed by palladium withdrawing the oxygen. The adsorbed carbon monoxide on palladium undergoes oxidation to form carbon dioxide, which then migrates to the this compound surface and desorbs. [] [https://www.semanticscholar.org/paper/a5a1d2772c69534bf75716cbacc988b69bd0028f]
- Decomposition of nitric oxide: this compound catalyzes the reaction between ethane and nitric oxide, yielding nitrogen, carbon dioxide, nitrous oxide, and water. The mechanism is thought to be complex, potentially involving surface nitrate intermediates. [] [https://www.semanticscholar.org/paper/db05dad3edaaddb5845a8ac21d2d018f6a8988c3]
- Oxygen evolution reaction: Calcium manganese(III) oxides, with compositions and structures mimicking the active site of photosystem II, display promising catalytic activity for water oxidation to molecular oxygen. [, ] [https://www.semanticscholar.org/paper/161806d2f8fa92bf582e2306001aecc3da5219c8, https://www.semanticscholar.org/paper/043c3e2de98033520a69f1582189a7d26d6139a1]
Q4: How does the catalytic activity of this compound compare to other transition metal oxides?
A4: In the context of 1-butene oxidation by nitrous oxide, the catalytic activity of various transition metal oxides follows this order: CuO > Fe2O3 > NiO > Mn2O3 > Cr2O3 > TiO2 > Sc2O3 > SnO > ZnO > V2O5 > Al2O3. This suggests that copper(II) oxide exhibits the highest activity, while this compound falls somewhere in the middle of the series. [] [https://www.semanticscholar.org/paper/de25737870fc689ff837e4acdbd75e26d617e8cc]
Q5: What are some potential applications of this compound's catalytic properties?
A5: The catalytic properties of this compound make it potentially valuable in a range of applications such as:
- Environmental remediation: Its ability to catalyze the oxidation of hydrocarbons and carbon monoxide could be harnessed for removing these pollutants from exhaust gases. [, , ] [https://www.semanticscholar.org/paper/a5a1d2772c69534bf75716cbacc988b69bd0028f, https://www.semanticscholar.org/paper/fbbecfada0598608758f6b628d0bb19272594a37, https://www.semanticscholar.org/paper/de25737870fc689ff837e4acdbd75e26d617e8cc]
- Chemical synthesis: Its ability to mediate organic reactions, such as the formation of tetrahydrofuran derivatives and the acetonylation of alkenes, highlights its potential as a catalyst in organic synthesis. [] [https://www.semanticscholar.org/paper/433bd7a2e92504676d22c07e4f005f3e5217df9c]
- Energy production and storage: Its role in catalyzing the oxygen evolution reaction is critical for developing efficient energy storage and conversion devices, such as fuel cells and electrolyzers. [, ] [https://www.semanticscholar.org/paper/161806d2f8fa92bf582e2306001aecc3da5219c8, https://www.semanticscholar.org/paper/043c3e2de98033520a69f1582189a7d26d6139a1]
Q6: How does this compound behave under different environmental conditions, particularly in water treatment?
A6: this compound plays a significant role in the removal of dissolved manganese in water treatment clarification processes. It acts as an adsorbent for manganese(II) ions, and this adsorption is influenced by factors such as pH, iron and manganese concentrations, and ionic strength. At higher pH levels, adsorbed manganese on the iron(III) oxide surface undergoes oxidation, potentially forming this compound. [] [https://www.semanticscholar.org/paper/1a2e6a2c95bf1d6442ab33c2e890da4a98f2dd5a]
Q7: What is the role of this compound in passive remediation systems for metal-polluted water?
A7: Caustic magnesia (magnesium oxide) reacts with water to form magnesium hydroxide, raising the pH. This facilitates the precipitation of metals like zinc, lead, and copper as hydroxides or hydroxysulfates. In this environment, manganese(II) undergoes oxidation and precipitates as this compound, effectively removing it from the water. [] [https://www.semanticscholar.org/paper/487d3fb0982a55f84b2b3d73293b7a7b9c43351e]
Q8: How is this compound used in analytical chemistry?
A8: this compound finds application in analytical chemistry for the determination of dissolved inorganic carbon (DIC) in seawater. It acts as an electroactive material in electrodes for electrolytic acidification, offering a reagentless method for pH modification in in situ ocean analysis. [] [https://www.semanticscholar.org/paper/337b8148ed7eb555d53a082c9fc32a34c1210870]
Q9: What is the role of this compound in the development of novel materials for electrochemical applications?
A9: this compound has been explored in various electrochemical applications:
- Electrochemical synthesis: It shows potential as a catalyst for the anodic generation of hydrogen peroxide during water oxidation. [] [https://www.semanticscholar.org/paper/5c16072bb2724f38b660d39cddb7505f30721789]
- Electrode material: In conjunction with silver nanofibers, it facilitates direct electron transfer of hemoglobin, paving the way for sensitive biosensor development. [] [https://www.semanticscholar.org/paper/535389b2085adcc7d948cc2366601d92103f2a89]
Q10: What are the environmental considerations related to the use and disposal of this compound?
A10: While manganese is an essential micronutrient, excessive manganese exposure can be harmful. Research is needed to fully understand the environmental impact and degradation pathways of this compound, especially in the context of its applications in water treatment and other fields. Implementing responsible waste management and exploring recycling strategies for this compound are crucial for minimizing potential environmental risks. [] [https://www.semanticscholar.org/paper/5ee821a06757bd8b3d21eeed70a212ed08e87faf]
Avertissement et informations sur les produits de recherche in vitro
Veuillez noter que tous les articles et informations sur les produits présentés sur BenchChem sont destinés uniquement à des fins informatives. Les produits disponibles à l'achat sur BenchChem sont spécifiquement conçus pour des études in vitro, qui sont réalisées en dehors des organismes vivants. Les études in vitro, dérivées du terme latin "in verre", impliquent des expériences réalisées dans des environnements de laboratoire contrôlés à l'aide de cellules ou de tissus. Il est important de noter que ces produits ne sont pas classés comme médicaments et n'ont pas reçu l'approbation de la FDA pour la prévention, le traitement ou la guérison de toute condition médicale, affection ou maladie. Nous devons souligner que toute forme d'introduction corporelle de ces produits chez les humains ou les animaux est strictement interdite par la loi. Il est essentiel de respecter ces directives pour assurer la conformité aux normes légales et éthiques en matière de recherche et d'expérimentation.