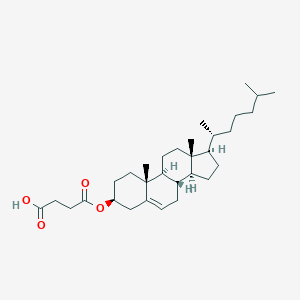
Cholesteryl hemisuccinate
Vue d'ensemble
Description
L'hémisuccinate de cholestéryle est un monoester d'acide dicarboxylique résultant de la condensation formelle du groupe hydroxyle du cholestérol avec l'un des groupes carboxyliques de l'acide succinique . Il est souvent utilisé comme détergent pour remplacer le cholestérol en cristallographie protéique, en études biochimiques de protéines et en pharmacologie . L'hémisuccinate de cholestéryle est connu pour sa capacité à stabiliser les grandes vésicules unilamellaires, ce qui en fait un composant précieux dans diverses applications scientifiques et industrielles .
Mécanisme D'action
Target of Action
Cholesteryl hemisuccinate (CHEMS) primarily targets cell membranes and DNA polymerase . It is often used to replace cholesterol in the crystallization of membrane proteins . It also inhibits DNA polymerase, a key enzyme involved in DNA replication and repair .
Mode of Action
CHEMS interacts with cell membranes and DNA polymerase to exert its effects. In cell membranes, CHEMS mimics many of the properties of cholesterol, particularly in saturated protein-free lipid membranes . The protonated form of CHEMS closely resembles cholesterol, while the deprotonated form is less similar . This mimicry allows CHEMS to replace cholesterol in certain biochemical processes, such as the crystallization of membrane proteins .
In terms of DNA polymerase, CHEMS acts as an inhibitor, thereby suppressing DNA replication and repair, as well as cell division .
Biochemical Pathways
The primary biochemical pathway affected by CHEMS is the DNA replication and repair pathway . By inhibiting DNA polymerase, CHEMS disrupts the normal functioning of this pathway, leading to a decrease in DNA replication and repair, and ultimately, cell division .
Result of Action
The inhibition of DNA polymerase by CHEMS leads to a decrease in DNA replication and repair, and cell division . This can result in antiproliferative effects , particularly in the context of cancer cells . CHEMS also has hepatoprotective effects, as it can inhibit the hepatotoxicity of acetaminophen (AAP) and prevent AAP-induced apoptosis and necrosis in liver cells .
Action Environment
The action of CHEMS is influenced by the pH of its environment. CHEMS exhibits pH-sensitive polymorphism, meaning its structure and behavior can change depending on the pH . In alkaline and neutral aqueous media, CHEMS can self-assemble into bilayers, mimicking the behavior of cholesterol .
Analyse Biochimique
Biochemical Properties
Cholesteryl hemisuccinate interacts with various biomolecules in biochemical reactions. It self-assembles into bilayers in alkaline and neutral aqueous media . It exhibits pH-sensitive polymorphism and consists of succinic acid esterified to the beta-hydroxyl group of cholesterol . It is also known to inhibit DNA polymerase and DNA topoisomerase, thereby inhibiting DNA replication and repair, as well as cell division .
Cellular Effects
This compound has significant effects on various types of cells and cellular processes. It is an oxidation product of polyunsaturated fatty acid of cholesterol that alters lysosome structure and functions and induces proinflammatory cytokine production in macrophages . It also inhibits DNA polymerase and DNA topoisomerase, thereby inhibiting DNA replication and repair, as well as cell division .
Molecular Mechanism
The molecular mechanism of action of this compound is primarily rooted in its interactions with cell membranes. By interacting with the membrane, this compound can induce alterations in permeability and fluidity, subsequently impacting the activity of embedded proteins, enzymes, and hormones .
Temporal Effects in Laboratory Settings
In laboratory settings, the effects of this compound change over time. For instance, it has been observed that the protonated form of this compound mimics many of the membrane properties of cholesterol quite well, while the deprotonated form is less convincing . This suggests that the effects of this compound on cellular function may vary over time, depending on its state and the conditions of the experiment .
Méthodes De Préparation
Voies de synthèse et conditions réactionnelles
La préparation de l'hémisuccinate de cholestéryle implique l'estérification du cholestérol avec l'anhydride succinique. La réaction nécessite généralement un catalyseur, tel que la pyridine, et est effectuée sous reflux. Le produit obtenu est purifié par recristallisation ou chromatographie pour obtenir de l'hémisuccinate de cholestéryle pur .
Méthodes de production industrielle
En milieu industriel, l'hémisuccinate de cholestéryle est produit en l'incorporant dans des solutions mères de détergent. Le procédé implique la solubilisation du détergent sec dans une solution tampon, suivie de l'ajout d'hémisuccinate de cholestéryle. Le mélange est ensuite soniqué et mélangé jusqu'à obtenir une distribution homogène .
Analyse Des Réactions Chimiques
Types de réactions
L'hémisuccinate de cholestéryle subit diverses réactions chimiques, notamment :
Oxydation : L'hémisuccinate de cholestéryle peut être oxydé pour former du peroxyde d'hémisuccinate de cholestéryle.
Réduction : Les réactions de réduction peuvent convertir l'hémisuccinate de cholestéryle en cholestérol.
Substitution : Le groupe ester de l'hémisuccinate de cholestéryle peut être substitué par d'autres groupes fonctionnels.
Réactifs et conditions courants
Oxydation : Les agents oxydants courants comprennent le peroxyde d'hydrogène et le permanganate de potassium.
Réduction : Des agents réducteurs tels que l'hydrure de lithium et d'aluminium sont utilisés.
Substitution : Divers nucléophiles peuvent être utilisés pour les réactions de substitution, en fonction du produit souhaité.
Principaux produits formés
Oxydation : Peroxyde d'hémisuccinate de cholestéryle.
Réduction : Cholestérol.
Substitution : Divers dérivés du cholestérol en fonction du nucléophile utilisé.
Applications De Recherche Scientifique
Pharmaceutical Applications
1.1 Drug Delivery Systems
Cholesteryl hemisuccinate is widely used in the formulation of liposomal drug delivery systems. Its incorporation into lipid bilayers enhances the stability and encapsulation efficiency of therapeutic agents. CHEMS-modified liposomes have shown improved circulation times and reduced clearance by the reticuloendothelial system, making them suitable for delivering drugs in vivo .
1.2 pH-Sensitive Liposomes
Research indicates that CHEMS can form pH-sensitive liposomes, which release their payload in response to acidic environments, such as those found in tumor tissues or inflamed areas . This property is particularly useful for targeted cancer therapies.
1.3 Membrane Stabilization
CHEMS acts as a membrane stabilizer in liposomal formulations, enhancing the structural integrity of lipid membranes under various physiological conditions. Studies have demonstrated that CHEMS can improve the stability of dipalmitoylphosphatidylcholine (DPPC) liposomes, which are commonly used in drug delivery applications .
Biophysical Studies
2.1 Membrane Biophysics
this compound has been utilized in biophysical studies to understand lipid bilayer dynamics and properties. Its presence affects the phase behavior of lipid membranes, influencing their fluidity and permeability . Research has shown that CHEMS can modulate calcium phosphate interactions at the air-liquid interface, providing insights into membrane interactions with ions .
2.2 Protein Crystallography
In biochemical studies, CHEMS serves as a cholesterol substitute in protein crystallography. It aids in stabilizing membrane proteins, allowing for better crystallization conditions and improved structural analysis . This application is crucial for understanding protein functions and interactions at a molecular level.
Nanotechnology
3.1 Lipid-Based Nano-Carriers
CHEMS is employed in the development of lipid-based nano-carriers for various biomedical applications, including gene delivery and vaccine formulations. Its ability to form stable nanoparticles enhances the delivery efficiency of nucleic acids and antigens . The incorporation of CHEMS into these carriers improves their biocompatibility and reduces immunogenic responses.
3.2 Skin Care Formulations
Recent studies have explored the use of this compound in skin-care products due to its emulsifying properties and ability to enhance skin penetration of active ingredients. Formulations containing CHEMS have shown promise in improving the delivery of hydrophilic compounds across the skin barrier .
Case Studies
Comparaison Avec Des Composés Similaires
Composés similaires
Cholestérol : Le composé parent de l'hémisuccinate de cholestéryle, largement connu pour son rôle dans les membranes cellulaires.
Oléate de cholestéryle : Un autre ester de cholestérol, utilisé dans des applications similaires mais avec des propriétés différentes.
Palmitate de cholestéryle : Un ester de cholestérol avec des propriétés physiques et chimiques distinctes.
Unicité
L'hémisuccinate de cholestéryle est unique en raison de sa capacité à former des vésicules stables et de son polymorphisme sensible au pH. Il est particulièrement précieux dans la cristallisation des protéines membranaires et comme agent de délivrance dans les formulations de médicaments .
Activité Biologique
Cholesteryl hemisuccinate (CHEMS) is a cholesterol derivative that has garnered attention for its unique biological properties and applications in various fields, including drug delivery and membrane biophysics. This article explores the biological activity of CHEMS, supported by research findings, case studies, and data tables.
This compound is an ester formed from cholesterol and succinic acid. Its chemical formula is with a molecular weight of 486.732 g/mol. The presence of a carboxylic group in CHEMS imparts surfactant-like properties, making it useful in the formation of liposomes and other lipid-based delivery systems.
Biophysical Properties
CHEMS exhibits pH-sensitive polymorphism , which is crucial for its application in drug delivery systems. Research indicates that CHEMS can self-assemble into bilayers in neutral to alkaline conditions while undergoing significant structural changes in acidic environments. This property enhances its ability to form fusogenic vesicles when mixed with other lipids, such as dioleoylphosphatidylethanolamine (DOPE) .
Table 1: Summary of Biophysical Properties of this compound
Property | Description |
---|---|
Molecular Weight | 486.732 g/mol |
pH Sensitivity | Exhibits phase changes at pH < 4.3 |
Self-Assembly | Forms bilayers in neutral/alkaline media |
Fusogenic Capability | Enhances lipid mixing and fusion in vesicular systems |
1. Drug Delivery Systems
CHEMS has been extensively studied for its role in enhancing the delivery of therapeutic agents. Its ability to form stable liposomes allows for improved encapsulation and release profiles of drugs. For instance, studies have shown that CHEMS can facilitate the delivery of hydrophilic drugs through lipid-based carriers, improving their bioavailability .
2. Gene Delivery
In gene therapy applications, CHEMS has been incorporated into cationic liposomes to enhance the transfection efficiency of nucleic acids. A study demonstrated that CHEMS-modified liposomes could effectively deliver plasmid DNA into cells, overcoming barriers posed by extracellular matrix components .
3. Membrane Fluidity Studies
Research involving pea chloroplast thylakoids has shown that incorporating CHEMS affects membrane fluidity, which is critical for photosynthetic efficiency. Increased sterol content from CHEMS was found to enhance membrane viscosity, influencing the functional dynamics of thylakoids .
Case Study 1: pH-Sensitive Liposomes
A study investigated the fusogenic properties of CHEMS when mixed with DOPE to create pH-sensitive liposomes. These liposomes demonstrated significant fusion capabilities at acidic pH levels, making them suitable for targeted drug delivery applications where local acidity is prevalent (e.g., tumor microenvironments) .
Case Study 2: Skin Penetration Enhancement
In skin penetration studies, CHEMS was evaluated for its ability to enhance the permeation of therapeutic compounds through the stratum corneum. The results indicated that formulations containing CHEMS significantly improved the delivery of model compounds compared to controls without CHEMS .
Research Findings
Recent studies have highlighted several key findings regarding the biological activity of this compound:
- Fusogenic Properties : CHEMS enhances lipid mixing and fusion in vesicular systems, crucial for drug delivery applications .
- Gene Delivery Efficiency : Incorporation into cationic liposomes increases transfection rates of plasmid DNA .
- Membrane Dynamics : Alters membrane viscosity and fluidity, impacting cellular processes such as photosynthesis .
Propriétés
IUPAC Name |
4-[[(3S,8S,9S,10R,13R,14S,17R)-10,13-dimethyl-17-[(2R)-6-methylheptan-2-yl]-2,3,4,7,8,9,11,12,14,15,16,17-dodecahydro-1H-cyclopenta[a]phenanthren-3-yl]oxy]-4-oxobutanoic acid | |
---|---|---|
Source | PubChem | |
URL | https://pubchem.ncbi.nlm.nih.gov | |
Description | Data deposited in or computed by PubChem | |
InChI |
InChI=1S/C31H50O4/c1-20(2)7-6-8-21(3)25-11-12-26-24-10-9-22-19-23(35-29(34)14-13-28(32)33)15-17-30(22,4)27(24)16-18-31(25,26)5/h9,20-21,23-27H,6-8,10-19H2,1-5H3,(H,32,33)/t21-,23+,24+,25-,26+,27+,30+,31-/m1/s1 | |
Source | PubChem | |
URL | https://pubchem.ncbi.nlm.nih.gov | |
Description | Data deposited in or computed by PubChem | |
InChI Key |
WLNARFZDISHUGS-MIXBDBMTSA-N | |
Source | PubChem | |
URL | https://pubchem.ncbi.nlm.nih.gov | |
Description | Data deposited in or computed by PubChem | |
Canonical SMILES |
CC(C)CCCC(C)C1CCC2C1(CCC3C2CC=C4C3(CCC(C4)OC(=O)CCC(=O)O)C)C | |
Source | PubChem | |
URL | https://pubchem.ncbi.nlm.nih.gov | |
Description | Data deposited in or computed by PubChem | |
Isomeric SMILES |
C[C@H](CCCC(C)C)[C@H]1CC[C@@H]2[C@@]1(CC[C@H]3[C@H]2CC=C4[C@@]3(CC[C@@H](C4)OC(=O)CCC(=O)O)C)C | |
Source | PubChem | |
URL | https://pubchem.ncbi.nlm.nih.gov | |
Description | Data deposited in or computed by PubChem | |
Molecular Formula |
C31H50O4 | |
Source | PubChem | |
URL | https://pubchem.ncbi.nlm.nih.gov | |
Description | Data deposited in or computed by PubChem | |
DSSTOX Substance ID |
DTXSID10934145 | |
Record name | 4-[(Cholest-5-en-3-yl)oxy]-4-oxobutanoic acid | |
Source | EPA DSSTox | |
URL | https://comptox.epa.gov/dashboard/DTXSID10934145 | |
Description | DSSTox provides a high quality public chemistry resource for supporting improved predictive toxicology. | |
Molecular Weight |
486.7 g/mol | |
Source | PubChem | |
URL | https://pubchem.ncbi.nlm.nih.gov | |
Description | Data deposited in or computed by PubChem | |
CAS No. |
1510-21-0 | |
Record name | Cholesteryl hemisuccinate | |
Source | CAS Common Chemistry | |
URL | https://commonchemistry.cas.org/detail?cas_rn=1510-21-0 | |
Description | CAS Common Chemistry is an open community resource for accessing chemical information. Nearly 500,000 chemical substances from CAS REGISTRY cover areas of community interest, including common and frequently regulated chemicals, and those relevant to high school and undergraduate chemistry classes. This chemical information, curated by our expert scientists, is provided in alignment with our mission as a division of the American Chemical Society. | |
Explanation | The data from CAS Common Chemistry is provided under a CC-BY-NC 4.0 license, unless otherwise stated. | |
Record name | Cholesteryl succinate | |
Source | ChemIDplus | |
URL | https://pubchem.ncbi.nlm.nih.gov/substance/?source=chemidplus&sourceid=0001510210 | |
Description | ChemIDplus is a free, web search system that provides access to the structure and nomenclature authority files used for the identification of chemical substances cited in National Library of Medicine (NLM) databases, including the TOXNET system. | |
Record name | 4-[(Cholest-5-en-3-yl)oxy]-4-oxobutanoic acid | |
Source | EPA DSSTox | |
URL | https://comptox.epa.gov/dashboard/DTXSID10934145 | |
Description | DSSTox provides a high quality public chemistry resource for supporting improved predictive toxicology. | |
Record name | Cholest-5-en-3β-yl hydrogen succinate | |
Source | European Chemicals Agency (ECHA) | |
URL | https://echa.europa.eu/substance-information/-/substanceinfo/100.014.680 | |
Description | The European Chemicals Agency (ECHA) is an agency of the European Union which is the driving force among regulatory authorities in implementing the EU's groundbreaking chemicals legislation for the benefit of human health and the environment as well as for innovation and competitiveness. | |
Explanation | Use of the information, documents and data from the ECHA website is subject to the terms and conditions of this Legal Notice, and subject to other binding limitations provided for under applicable law, the information, documents and data made available on the ECHA website may be reproduced, distributed and/or used, totally or in part, for non-commercial purposes provided that ECHA is acknowledged as the source: "Source: European Chemicals Agency, http://echa.europa.eu/". Such acknowledgement must be included in each copy of the material. ECHA permits and encourages organisations and individuals to create links to the ECHA website under the following cumulative conditions: Links can only be made to webpages that provide a link to the Legal Notice page. | |
Record name | CHOLESTERYL SUCCINATE | |
Source | FDA Global Substance Registration System (GSRS) | |
URL | https://gsrs.ncats.nih.gov/ginas/app/beta/substances/T3J4KS4201 | |
Description | The FDA Global Substance Registration System (GSRS) enables the efficient and accurate exchange of information on what substances are in regulated products. Instead of relying on names, which vary across regulatory domains, countries, and regions, the GSRS knowledge base makes it possible for substances to be defined by standardized, scientific descriptions. | |
Explanation | Unless otherwise noted, the contents of the FDA website (www.fda.gov), both text and graphics, are not copyrighted. They are in the public domain and may be republished, reprinted and otherwise used freely by anyone without the need to obtain permission from FDA. Credit to the U.S. Food and Drug Administration as the source is appreciated but not required. | |
Retrosynthesis Analysis
AI-Powered Synthesis Planning: Our tool employs the Template_relevance Pistachio, Template_relevance Bkms_metabolic, Template_relevance Pistachio_ringbreaker, Template_relevance Reaxys, Template_relevance Reaxys_biocatalysis model, leveraging a vast database of chemical reactions to predict feasible synthetic routes.
One-Step Synthesis Focus: Specifically designed for one-step synthesis, it provides concise and direct routes for your target compounds, streamlining the synthesis process.
Accurate Predictions: Utilizing the extensive PISTACHIO, BKMS_METABOLIC, PISTACHIO_RINGBREAKER, REAXYS, REAXYS_BIOCATALYSIS database, our tool offers high-accuracy predictions, reflecting the latest in chemical research and data.
Strategy Settings
Precursor scoring | Relevance Heuristic |
---|---|
Min. plausibility | 0.01 |
Model | Template_relevance |
Template Set | Pistachio/Bkms_metabolic/Pistachio_ringbreaker/Reaxys/Reaxys_biocatalysis |
Top-N result to add to graph | 6 |
Feasible Synthetic Routes
Avertissement et informations sur les produits de recherche in vitro
Veuillez noter que tous les articles et informations sur les produits présentés sur BenchChem sont destinés uniquement à des fins informatives. Les produits disponibles à l'achat sur BenchChem sont spécifiquement conçus pour des études in vitro, qui sont réalisées en dehors des organismes vivants. Les études in vitro, dérivées du terme latin "in verre", impliquent des expériences réalisées dans des environnements de laboratoire contrôlés à l'aide de cellules ou de tissus. Il est important de noter que ces produits ne sont pas classés comme médicaments et n'ont pas reçu l'approbation de la FDA pour la prévention, le traitement ou la guérison de toute condition médicale, affection ou maladie. Nous devons souligner que toute forme d'introduction corporelle de ces produits chez les humains ou les animaux est strictement interdite par la loi. Il est essentiel de respecter ces directives pour assurer la conformité aux normes légales et éthiques en matière de recherche et d'expérimentation.