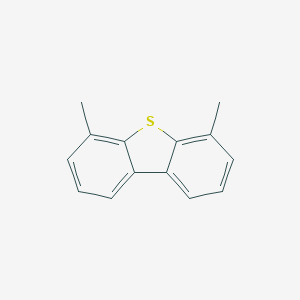
4,6-Dimethyldibenzothiophene
Vue d'ensemble
Description
4,6-Dimethyldibenzothiophene is an organosulfur compound with the formula (C6H3CH3)2S . It is one of several dimethyl derivatives of benzothiophene . The compound is of particular interest as an organosulfur contaminant in petroleum that is recalcitrant . Both methyl groups shield the sulfur center from desulfurization .
Synthesis Analysis
The synthesis of 4,6-Dimethyldibenzothiophene has been reported . The compound can be oxidized to the sulfoxide with hydrogen peroxide . Many methods for synthesis of this compound have been described. Often the methods proceed via derivatives of diphenyl sulfide .Molecular Structure Analysis
The molecular structure of 4,6-Dimethyldibenzothiophene is available as a 2D Mol file or as a computed 3D SD file .Chemical Reactions Analysis
4,6-Dimethyldibenzothiophene can be oxidized to its corresponding sulfones by an Anderson-type catalyst using molecular oxygen as the oxidant under mild reaction conditions . The hydrodesulfurization of 4,6-Dimethyldibenzothiophene using bulk nickel alloy, bulk tungsten phosphide (WP), NiMo sulfide supported on active carbons and molecularly imprinted polymers have been reported .Physical And Chemical Properties Analysis
4,6-Dimethyldibenzothiophene is a light yellow to light brown crystalline solid . It has a molar mass of 212.31 g·mol −1 and a density of 1.18 g/cm 3 . The melting point is 153–157 °C .Applications De Recherche Scientifique
Desulfurization of Fuels
4,6-Dimethyldibenzothiophene is used in the study of desulfurization of fuels . The burning of sulfur-containing fuels leads to the generation of harmful compounds which are a major source of environmental pollution . Adsorptive desulfurization (ADS) is a method that has low energy demand and can be employed at ambient conditions without the addition of chemicals . Metal Organic Frameworks (MOFs) have proved to be very effective towards this direction .
Adsorption Studies
The compound is used in adsorption studies . The adsorption of BT, DBT, and 4,6-dimethyldibenzothiophene using different MOFs under varying experimental conditions has been studied . HKUST-1, a benchmark MOF, is especially used for gaseous adsorption-oriented applications .
Simultaneous Adsorption with Quinoline
4,6-Dimethyldibenzothiophene is used in the study of simultaneous adsorption of quinoline . The adsorbents used are based on alumina modified with boron and nickel under ambient temperature and pressure . The adsorbent containing nickel had better adsorption capacity than the adsorbent modified with nickel and boron .
Hydrodesulfurization Studies
The compound is used in hydrodesulfurization (HDS) studies . Molecules such as 4,6-dimethyldibenzothiophene, with alkyl groups adjacent to the sulfur atom, are most difficult to desulfurize and are therefore often used as model molecules in deep HDS studies .
Petroleum Desulfurization Studies
4,6-Dimethyldibenzothiophene is used in petroleum desulfurization studies . This compound is part of the Alfa Aesar product portfolio, which is now a Thermo Scientific Chemicals brand product .
Environmental Pollution Studies
The compound is used in environmental pollution studies . The emission of hazardous and dangerous chemical substances during the use of fossil fuels is an important threat to human society as well as the environment . The study of these emissions involves the use of 4,6-Dimethyldibenzothiophene .
Mécanisme D'action
Target of Action
4,6-Dimethyldibenzothiophene (4,6-DMDBT) is an organosulfur compound . It is of particular interest as an organosulfur contaminant in petroleum that is recalcitrant . The primary targets of 4,6-DMDBT are the sulfur centers in the compound, which are shielded by the two methyl groups .
Mode of Action
The compound interacts with its targets primarily through desulfurization processes . The two methyl groups shield the sulfur center from desulfurization . The compound can be oxidized to the sulfoxide with hydrogen peroxide .
Biochemical Pathways
The primary biochemical pathway affected by 4,6-DMDBT is the desulfurization process . The compound’s resistance to desulfurization makes it a significant challenge in the petroleum industry .
Pharmacokinetics
Its physical properties, such as its density (118 g/cm^3) and melting point (153–157 °C), may influence its behavior in an industrial setting .
Result of Action
The resistance of 4,6-DMDBT to desulfurization results in its persistence in petroleum products . This resistance is due to the shielding of the sulfur center by the two methyl groups .
Safety and Hazards
4,6-Dimethyldibenzothiophene is harmful if swallowed . It is advised to avoid breathing mist, gas or vapours, avoid contacting with skin and eye, use personal protective equipment, wear chemical impermeable gloves, ensure adequate ventilation, remove all sources of ignition, evacuate personnel to safe areas, and keep people away from and upwind of spill/leak .
Orientations Futures
In the future, the hydrodesulfurization of 4,6-Dimethyldibenzothiophene could be performed under lower conditions than conventional hydrotreating processes . The use of n–dodecane as a solvent decreased the mass transfer resistance at the G–L and liquid–solid (L–S) interphases . The catalytic activity improved when the acidity and surface area in the catalysts increased .
Propriétés
IUPAC Name |
4,6-dimethyldibenzothiophene | |
---|---|---|
Source | PubChem | |
URL | https://pubchem.ncbi.nlm.nih.gov | |
Description | Data deposited in or computed by PubChem | |
InChI |
InChI=1S/C14H12S/c1-9-5-3-7-11-12-8-4-6-10(2)14(12)15-13(9)11/h3-8H,1-2H3 | |
Source | PubChem | |
URL | https://pubchem.ncbi.nlm.nih.gov | |
Description | Data deposited in or computed by PubChem | |
InChI Key |
MYAQZIAVOLKEGW-UHFFFAOYSA-N | |
Source | PubChem | |
URL | https://pubchem.ncbi.nlm.nih.gov | |
Description | Data deposited in or computed by PubChem | |
Canonical SMILES |
CC1=C2C(=CC=C1)C3=CC=CC(=C3S2)C | |
Source | PubChem | |
URL | https://pubchem.ncbi.nlm.nih.gov | |
Description | Data deposited in or computed by PubChem | |
Molecular Formula |
C14H12S | |
Source | PubChem | |
URL | https://pubchem.ncbi.nlm.nih.gov | |
Description | Data deposited in or computed by PubChem | |
Molecular Weight |
212.31 g/mol | |
Source | PubChem | |
URL | https://pubchem.ncbi.nlm.nih.gov | |
Description | Data deposited in or computed by PubChem | |
CAS RN |
1207-12-1 | |
Record name | 4,6-Dimethyldibenzothiophene | |
Source | CAS Common Chemistry | |
URL | https://commonchemistry.cas.org/detail?cas_rn=1207-12-1 | |
Description | CAS Common Chemistry is an open community resource for accessing chemical information. Nearly 500,000 chemical substances from CAS REGISTRY cover areas of community interest, including common and frequently regulated chemicals, and those relevant to high school and undergraduate chemistry classes. This chemical information, curated by our expert scientists, is provided in alignment with our mission as a division of the American Chemical Society. | |
Explanation | The data from CAS Common Chemistry is provided under a CC-BY-NC 4.0 license, unless otherwise stated. | |
Record name | Dibenzothiophene, 4,6-dimethyl- | |
Source | EPA Chemicals under the TSCA | |
URL | https://www.epa.gov/chemicals-under-tsca | |
Description | EPA Chemicals under the Toxic Substances Control Act (TSCA) collection contains information on chemicals and their regulations under TSCA, including non-confidential content from the TSCA Chemical Substance Inventory and Chemical Data Reporting. | |
Record name | 4,6-dimethyldibenzothiophene | |
Source | European Chemicals Agency (ECHA) | |
URL | https://echa.europa.eu/substance-information/-/substanceinfo/100.013.540 | |
Description | The European Chemicals Agency (ECHA) is an agency of the European Union which is the driving force among regulatory authorities in implementing the EU's groundbreaking chemicals legislation for the benefit of human health and the environment as well as for innovation and competitiveness. | |
Explanation | Use of the information, documents and data from the ECHA website is subject to the terms and conditions of this Legal Notice, and subject to other binding limitations provided for under applicable law, the information, documents and data made available on the ECHA website may be reproduced, distributed and/or used, totally or in part, for non-commercial purposes provided that ECHA is acknowledged as the source: "Source: European Chemicals Agency, http://echa.europa.eu/". Such acknowledgement must be included in each copy of the material. ECHA permits and encourages organisations and individuals to create links to the ECHA website under the following cumulative conditions: Links can only be made to webpages that provide a link to the Legal Notice page. | |
Retrosynthesis Analysis
AI-Powered Synthesis Planning: Our tool employs the Template_relevance Pistachio, Template_relevance Bkms_metabolic, Template_relevance Pistachio_ringbreaker, Template_relevance Reaxys, Template_relevance Reaxys_biocatalysis model, leveraging a vast database of chemical reactions to predict feasible synthetic routes.
One-Step Synthesis Focus: Specifically designed for one-step synthesis, it provides concise and direct routes for your target compounds, streamlining the synthesis process.
Accurate Predictions: Utilizing the extensive PISTACHIO, BKMS_METABOLIC, PISTACHIO_RINGBREAKER, REAXYS, REAXYS_BIOCATALYSIS database, our tool offers high-accuracy predictions, reflecting the latest in chemical research and data.
Strategy Settings
Precursor scoring | Relevance Heuristic |
---|---|
Min. plausibility | 0.01 |
Model | Template_relevance |
Template Set | Pistachio/Bkms_metabolic/Pistachio_ringbreaker/Reaxys/Reaxys_biocatalysis |
Top-N result to add to graph | 6 |
Feasible Synthetic Routes
Avertissement et informations sur les produits de recherche in vitro
Veuillez noter que tous les articles et informations sur les produits présentés sur BenchChem sont destinés uniquement à des fins informatives. Les produits disponibles à l'achat sur BenchChem sont spécifiquement conçus pour des études in vitro, qui sont réalisées en dehors des organismes vivants. Les études in vitro, dérivées du terme latin "in verre", impliquent des expériences réalisées dans des environnements de laboratoire contrôlés à l'aide de cellules ou de tissus. Il est important de noter que ces produits ne sont pas classés comme médicaments et n'ont pas reçu l'approbation de la FDA pour la prévention, le traitement ou la guérison de toute condition médicale, affection ou maladie. Nous devons souligner que toute forme d'introduction corporelle de ces produits chez les humains ou les animaux est strictement interdite par la loi. Il est essentiel de respecter ces directives pour assurer la conformité aux normes légales et éthiques en matière de recherche et d'expérimentation.