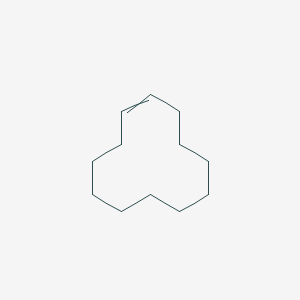
Cyclododécène, (E)-
Vue d'ensemble
Description
Cyclododecene is a cyclic hydrocarbon with the molecular formula C₁₂H₂₂. It is a twelve-membered ring containing one double bond, making it an unsaturated compound. Cyclododecene is known for its applications in the synthesis of various chemicals and materials, particularly in the polymer industry.
Applications De Recherche Scientifique
Cyclododecene has several applications in scientific research, including:
Mécanisme D'action
Mode of Action
It is known to participate in various organic synthesis reactions .
Biochemical Pathways
Cyclododecene, (E)- is involved in several biochemical pathways. It is an intermediate in the production of Nylon 12, polyesters, and synthetic lubricating oils . It is also used in the synthesis of flame retardants, detergents, and other chemicals .
Pharmacokinetics
It is known that it is insoluble in water but soluble in alcohol .
Result of Action
The molecular and cellular effects of Cyclododecene, (E)-'s action are largely dependent on its role in the specific biochemical pathway in which it is involved. For instance, in the production of Nylon 12, it serves as a precursor to laurolactam .
Action Environment
Environmental factors can influence the action, efficacy, and stability of Cyclododecene, (E)-. For example, it is stable under room temperature conditions . It is also known to be lipophilic and has the potential to bioaccumulate . It is usually present in the environment adsorbed on the surface of soil particles .
Méthodes De Préparation
Synthetic Routes and Reaction Conditions: Cyclododecene is typically prepared by the partial hydrogenation of cyclododecatriene. The process involves the selective hydrogenation of one of the three double bonds in cyclododecatriene using a suitable catalyst, such as palladium or nickel, under controlled conditions. The reaction is carried out at elevated temperatures and pressures to achieve the desired selectivity and yield .
Industrial Production Methods: In industrial settings, cyclododecene is produced through a similar hydrogenation process. The reaction is conducted in large-scale reactors with continuous monitoring of temperature, pressure, and hydrogen flow to ensure optimal conversion rates and product purity. The resulting cyclododecene is then purified through distillation or other separation techniques to remove any unreacted starting materials and by-products .
Analyse Des Réactions Chimiques
Types of Reactions: Cyclododecene undergoes various chemical reactions, including:
Oxidation: Cyclododecene can be oxidized to form cyclododecanone or cyclododecanol using oxidizing agents such as potassium permanganate or chromium trioxide.
Reduction: The double bond in cyclododecene can be reduced to form cyclododecane using hydrogen gas in the presence of a metal catalyst like palladium on carbon.
Common Reagents and Conditions:
Oxidation: Potassium permanganate in an aqueous medium at room temperature.
Reduction: Hydrogen gas with palladium on carbon catalyst at elevated temperatures and pressures.
Substitution: Chlorine or bromine gas at room temperature or slightly elevated temperatures.
Major Products Formed:
Oxidation: Cyclododecanone, cyclododecanol.
Reduction: Cyclododecane.
Substitution: Dihalogenated cyclododecene derivatives.
Comparaison Avec Des Composés Similaires
- Cyclooctene
- Cyclohexene
- Cyclodecene
Propriétés
IUPAC Name |
cyclododecene | |
---|---|---|
Source | PubChem | |
URL | https://pubchem.ncbi.nlm.nih.gov | |
Description | Data deposited in or computed by PubChem | |
InChI |
InChI=1S/C12H22/c1-2-4-6-8-10-12-11-9-7-5-3-1/h1-2H,3-12H2 | |
Source | PubChem | |
URL | https://pubchem.ncbi.nlm.nih.gov | |
Description | Data deposited in or computed by PubChem | |
InChI Key |
HYPABJGVBDSCIT-UHFFFAOYSA-N | |
Source | PubChem | |
URL | https://pubchem.ncbi.nlm.nih.gov | |
Description | Data deposited in or computed by PubChem | |
Canonical SMILES |
C1CCCCCC=CCCCC1 | |
Source | PubChem | |
URL | https://pubchem.ncbi.nlm.nih.gov | |
Description | Data deposited in or computed by PubChem | |
Molecular Formula |
C12H22 | |
Source | PubChem | |
URL | https://pubchem.ncbi.nlm.nih.gov | |
Description | Data deposited in or computed by PubChem | |
DSSTOX Substance ID |
DTXSID20880669 | |
Record name | cyclododecene, (e)- | |
Source | EPA DSSTox | |
URL | https://comptox.epa.gov/dashboard/DTXSID20880669 | |
Description | DSSTox provides a high quality public chemistry resource for supporting improved predictive toxicology. | |
Molecular Weight |
166.30 g/mol | |
Source | PubChem | |
URL | https://pubchem.ncbi.nlm.nih.gov | |
Description | Data deposited in or computed by PubChem | |
Physical Description |
Colorless liquid; [Alfa Aesar MSDS] | |
Record name | Cyclododecene | |
Source | Haz-Map, Information on Hazardous Chemicals and Occupational Diseases | |
URL | https://haz-map.com/Agents/20495 | |
Description | Haz-Map® is an occupational health database designed for health and safety professionals and for consumers seeking information about the adverse effects of workplace exposures to chemical and biological agents. | |
Explanation | Copyright (c) 2022 Haz-Map(R). All rights reserved. Unless otherwise indicated, all materials from Haz-Map are copyrighted by Haz-Map(R). No part of these materials, either text or image may be used for any purpose other than for personal use. Therefore, reproduction, modification, storage in a retrieval system or retransmission, in any form or by any means, electronic, mechanical or otherwise, for reasons other than personal use, is strictly prohibited without prior written permission. | |
CAS No. |
1486-75-5, 1501-82-2 | |
Record name | cyclododecene, (e)- | |
Source | EPA DSSTox | |
URL | https://comptox.epa.gov/dashboard/DTXSID20880669 | |
Description | DSSTox provides a high quality public chemistry resource for supporting improved predictive toxicology. | |
Record name | Cyclododecene | |
Source | European Chemicals Agency (ECHA) | |
URL | https://echa.europa.eu/substance-information/-/substanceinfo/100.014.652 | |
Description | The European Chemicals Agency (ECHA) is an agency of the European Union which is the driving force among regulatory authorities in implementing the EU's groundbreaking chemicals legislation for the benefit of human health and the environment as well as for innovation and competitiveness. | |
Explanation | Use of the information, documents and data from the ECHA website is subject to the terms and conditions of this Legal Notice, and subject to other binding limitations provided for under applicable law, the information, documents and data made available on the ECHA website may be reproduced, distributed and/or used, totally or in part, for non-commercial purposes provided that ECHA is acknowledged as the source: "Source: European Chemicals Agency, http://echa.europa.eu/". Such acknowledgement must be included in each copy of the material. ECHA permits and encourages organisations and individuals to create links to the ECHA website under the following cumulative conditions: Links can only be made to webpages that provide a link to the Legal Notice page. | |
Synthesis routes and methods I
Procedure details
Synthesis routes and methods II
Procedure details
Synthesis routes and methods III
Procedure details
Retrosynthesis Analysis
AI-Powered Synthesis Planning: Our tool employs the Template_relevance Pistachio, Template_relevance Bkms_metabolic, Template_relevance Pistachio_ringbreaker, Template_relevance Reaxys, Template_relevance Reaxys_biocatalysis model, leveraging a vast database of chemical reactions to predict feasible synthetic routes.
One-Step Synthesis Focus: Specifically designed for one-step synthesis, it provides concise and direct routes for your target compounds, streamlining the synthesis process.
Accurate Predictions: Utilizing the extensive PISTACHIO, BKMS_METABOLIC, PISTACHIO_RINGBREAKER, REAXYS, REAXYS_BIOCATALYSIS database, our tool offers high-accuracy predictions, reflecting the latest in chemical research and data.
Strategy Settings
Precursor scoring | Relevance Heuristic |
---|---|
Min. plausibility | 0.01 |
Model | Template_relevance |
Template Set | Pistachio/Bkms_metabolic/Pistachio_ringbreaker/Reaxys/Reaxys_biocatalysis |
Top-N result to add to graph | 6 |
Feasible Synthetic Routes
Q1: What is the molecular formula and weight of cyclododecene?
A1: Cyclododecene has the molecular formula C12H22 and a molecular weight of 166.3 g/mol.
Q2: What spectroscopic techniques are useful for characterizing cyclododecene?
A2: Several spectroscopic techniques are commonly employed to characterize cyclododecene, including:
- NMR spectroscopy: Both 1H and 13C NMR are valuable for determining the structure and conformation of cyclododecene and its derivatives. [, , ]
- IR spectroscopy: Infrared spectroscopy is helpful in identifying functional groups, particularly the presence of the C=C double bond in cyclododecene. [, , ]
- UV-Vis spectroscopy: While not as commonly used for cyclododecene itself, UV-Vis spectroscopy is helpful in characterizing conjugated derivatives like dehydrobenzoannulenes. [, ]
Q3: What is the significance of cyclododecene in catalytic reactions?
A4: Cyclododecene serves as a valuable starting material and intermediate in various chemical syntheses. For example, it can be converted to 1,12-dodecanedioic acid, a precursor for nylon production, through ozonolysis. [] It's also used in the production of laurolactam, another nylon precursor. []
Q4: Can cyclododecene be selectively hydrogenated?
A5: Yes, cyclododecene can be selectively hydrogenated to cyclododecane. Research has focused on developing catalysts and reaction conditions that favor cyclododecene formation over complete hydrogenation to cyclododecane. One study investigated the use of palladium catalysts with regular pore systems for this purpose. [] Another study explored the use of bimetallic Ru-Sn nanoparticle catalysts for the selective hydrogenation of 1,5,9-cyclododecatriene to cyclododecene. []
Q5: How does the ring size of cycloalkenes influence their reactivity in epoxidation reactions?
A6: The reactivity of cycloalkenes in epoxidation reactions is significantly influenced by ring size. For instance, studies using iodosylbenzene and metalloporphyrin catalysts demonstrated that smaller rings like cyclopentene and cyclohexene are more prone to allylic oxidation, while larger rings like cyclooctene and cyclododecene primarily undergo epoxidation. This selectivity is attributed to the conformational constraints imposed by the ring size on the transition state of the allylic oxidation pathway. []
Q6: What are some examples of catalysts used for cyclododecene epoxidation?
A6: Various catalysts have been investigated for cyclododecene epoxidation, including:
- Titania-silica aerogels: These catalysts exhibit excellent activity and selectivity in cyclododecene epoxidation using cumene hydroperoxide as the oxidant. The drying method and calcination temperature significantly influence their performance. [, ]
- Tungsten-based catalysts: Tungsten oxide/2,2'-bipyridine hybrid materials have demonstrated high catalytic activity in cyclododecene epoxidation using aqueous hydrogen peroxide as the oxidant. These materials act as sources of soluble active species, specifically the oxodiperoxo complex, which forms in situ. []
Q7: Have computational methods been employed to study cyclododecene?
A8: Yes, computational chemistry techniques like molecular mechanics (MM) and quantum chemical calculations have been utilized to investigate the conformational behavior of cyclododecene. [, ] For example, MM calculations helped determine the most stable conformation of cis-cyclododecene. [, ] These methods have also been used to study the conformational preferences of trans-cyclododecene. []
Q8: Are there specific formulation strategies to improve the stability of cyclododecene?
A8: While cyclododecene is generally stable, specific formulation strategies may be employed to enhance its long-term stability, particularly if it's incorporated into a complex mixture or stored for extended periods. These strategies could include:* Storage under inert atmosphere: Storing cyclododecene under an inert atmosphere like nitrogen or argon minimizes the risk of oxidation.* Addition of antioxidants: Incorporating antioxidants into the formulation can help scavenge free radicals and prevent oxidative degradation.
Q9: What analytical methods are commonly used to quantify cyclododecene?
A10: Gas chromatography (GC) is widely used to quantify cyclododecene, often coupled with mass spectrometry (GC/MS) for accurate identification and quantification. []
Avertissement et informations sur les produits de recherche in vitro
Veuillez noter que tous les articles et informations sur les produits présentés sur BenchChem sont destinés uniquement à des fins informatives. Les produits disponibles à l'achat sur BenchChem sont spécifiquement conçus pour des études in vitro, qui sont réalisées en dehors des organismes vivants. Les études in vitro, dérivées du terme latin "in verre", impliquent des expériences réalisées dans des environnements de laboratoire contrôlés à l'aide de cellules ou de tissus. Il est important de noter que ces produits ne sont pas classés comme médicaments et n'ont pas reçu l'approbation de la FDA pour la prévention, le traitement ou la guérison de toute condition médicale, affection ou maladie. Nous devons souligner que toute forme d'introduction corporelle de ces produits chez les humains ou les animaux est strictement interdite par la loi. Il est essentiel de respecter ces directives pour assurer la conformité aux normes légales et éthiques en matière de recherche et d'expérimentation.