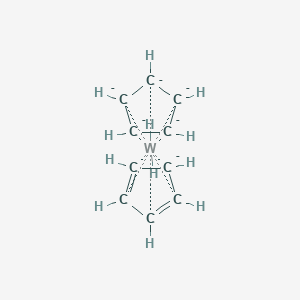
Cyclopenta-1,3-diene;tungsten(2+)
Vue d'ensemble
Description
Cyclopenta-1,3-diene;tungsten(2+) is an organometallic compound featuring a tungsten cation coordinated to cyclopentadienyl ligands. The compound is part of a broader class of metallocenes, where transition metals are sandwiched between cyclopentadienyl (Cp) rings. A structurally characterized example is 2-butylcyclopenta-1,3-diene;tungsten(2+), with the molecular formula C₁₈H₂₆W, a molecular weight of 426.24 g/mol, and a melting point of 83–87°C . Tungsten’s high oxidation state (+2) and large atomic radius distinguish it from lighter transition metals in similar complexes, influencing its electronic structure and reactivity. This compound is synthesized via ligand substitution or metathesis reactions, often involving tungsten halides and cyclopentadienyl precursors .
Méthodes De Préparation
Synthetic Routes and Reaction Conditions
The synthesis of cyclopenta-1,3-diene;tungsten(2+) typically involves the reaction of tungsten hexachloride (WCl6) with cyclopentadiene in the presence of a reducing agent. One common method is as follows:
Reduction of Tungsten Hexachloride: Tungsten hexachloride is reduced using a suitable reducing agent such as sodium or lithium in an inert atmosphere to prevent oxidation.
Formation of the Cyclopentadienyl Complex: The reduced tungsten species is then reacted with cyclopentadiene to form the cyclopenta-1,3-diene;tungsten(2+) complex.
The reaction is usually carried out in a solvent like tetrahydrofuran (THF) under an inert atmosphere (e.g., nitrogen or argon) to prevent the oxidation of the reactants and products.
Industrial Production Methods
Industrial production of cyclopenta-1,3-diene;tungsten(2+) follows similar principles but on a larger scale. The process involves:
Bulk Reduction: Large quantities of tungsten hexachloride are reduced using industrial-scale reducing agents.
Continuous Flow Reactors: The reduced tungsten is then continuously reacted with cyclopentadiene in flow reactors to ensure consistent product quality and yield.
Purification: The product is purified using techniques such as crystallization or chromatography to remove any impurities.
Analyse Des Réactions Chimiques
Types of Reactions
Cyclopenta-1,3-diene;tungsten(2+) undergoes various chemical reactions, including:
Oxidation: The compound can be oxidized to higher oxidation states of tungsten, often using oxidizing agents like oxygen or halogens.
Reduction: It can be reduced further to lower oxidation states or even to metallic tungsten using strong reducing agents.
Substitution: Ligand substitution reactions can occur where the cyclopentadienyl ligand is replaced by other ligands such as phosphines or carbonyls.
Common Reagents and Conditions
Oxidation: Oxygen, chlorine, or bromine under controlled conditions.
Reduction: Sodium, lithium, or potassium in an inert atmosphere.
Substitution: Phosphines (e.g., triphenylphosphine), carbonyls (e.g., carbon monoxide) in solvents like THF or dichloromethane.
Major Products
Oxidation: Higher oxidation state tungsten complexes.
Reduction: Lower oxidation state tungsten complexes or metallic tungsten.
Substitution: Various substituted tungsten complexes depending on the incoming ligand.
Applications De Recherche Scientifique
Catalytic Applications
Catalysis in Organic Reactions
Cyclopenta-1,3-diene;tungsten(2+) serves as an effective catalyst in multiple organic reactions:
- Hydrogenation : The compound facilitates the addition of hydrogen to unsaturated hydrocarbons, enhancing the yield of saturated products.
- Polymerization : It plays a crucial role in polymer synthesis, particularly in the formation of conductive polymers.
- Carbonylation : The compound is involved in carbonylation reactions, which are vital for producing aldehydes and acids from alkenes.
Mechanism of Action
The catalytic activity is attributed to the coordination of the tungsten atom with carbon monoxide and cyclopenta-1,3-diene ligands. This coordination alters the electronic structure of tungsten, enabling it to participate effectively in various chemical transformations.
Materials Science
Development of Advanced Materials
Cyclopenta-1,3-diene;tungsten(2+) is studied for its potential applications in materials science:
- Conductive Polymers : The compound is used to synthesize polymers with enhanced electrical conductivity, which are essential for electronic applications.
- Nanomaterials : Research indicates its utility in developing nanostructured materials that exhibit unique optical and electronic properties.
Case Study: Conductive Polymer Synthesis
A study demonstrated the successful use of cyclopenta-1,3-diene;tungsten(2+) in synthesizing a conductive polymer that exhibited improved charge transport properties compared to conventional materials. This advancement opens avenues for its application in organic electronics and sensors.
Biological Studies
Exploration of Biological Activity
Recent research has begun to investigate the biological implications of cyclopenta-1,3-diene;tungsten(2+):
- Drug Delivery Systems : The compound's ability to form stable complexes with biomolecules suggests potential applications in targeted drug delivery.
- Imaging Agents : Its unique properties may also facilitate the development of imaging agents for medical diagnostics.
Case Study: Drug Delivery Research
In a preliminary study, cyclopenta-1,3-diene;tungsten(2+) was evaluated for its efficacy as a drug delivery vehicle. Results indicated that it could encapsulate therapeutic agents effectively while providing controlled release profiles, making it a promising candidate for further exploration in pharmaceutical applications.
Mécanisme D'action
The mechanism by which cyclopenta-1,3-diene;tungsten(2+) exerts its effects involves the interaction of the tungsten center with various substrates. The cyclopentadienyl ligand stabilizes the tungsten ion, allowing it to participate in electron transfer reactions. The tungsten center can coordinate with different substrates, facilitating their activation and subsequent reaction. This coordination often involves the formation of transient intermediates that are crucial for the catalytic activity of the compound.
Comparaison Avec Des Composés Similaires
Comparison with Similar Compounds
Structural and Electronic Comparisons
Key Observations:
- Aromaticity : Unlike ferrocene, where the Cp ligands are fully aromatic, tungsten complexes may exhibit varied electron delocalization due to tungsten’s larger size and relativistic effects .
- Coordination Geometry : Tungsten’s higher oxidation state (+2 vs. Fe’s +2 or Ni’s +2) allows for diverse ligand environments, including halides or alkyl groups .
Physical and Chemical Properties
Reactivity Highlights:
- Tungsten Complexes : Exhibit higher thermal stability than ferrocene due to stronger metal-ligand bonds but are more reactive toward electrophiles due to tungsten’s electrophilic nature .
- Ferrocene : Undergoes facile Friedel-Crafts alkylation, while tungsten analogs may favor ligand substitution or redox reactions .
Research Findings and Trends
- Electronic Studies : ESR data for Cp cation radicals (e.g., H1,4 electron density = 1.16) suggest tungsten complexes may stabilize charge-separated states, useful in photoredox catalysis .
- Computational Insights : Density functional theory (DFT) studies on Cp-tungsten systems predict enhanced ligand-to-metal charge transfer compared to iron .
- Thermal Stability : Tungsten-Cp derivatives decompose above 200°C, outperforming nickel analogs (<150°C) .
Activité Biologique
Cyclopenta-1,3-diene; tungsten(2+) is an organometallic compound that has garnered attention in various fields due to its unique chemical properties and potential biological activities. This article provides a comprehensive overview of its biological activity, including synthesis methods, mechanisms of action, and relevant case studies.
Compound Overview
Chemical Structure and Properties
Cyclopenta-1,3-diene; tungsten(2+) is characterized by a tungsten atom coordinated to two cyclopenta-1,3-diene ligands. The compound's stability and reactivity are influenced by the unique bonding interactions between the metal and the diene ligands.
Property | Value |
---|---|
IUPAC Name | Cyclopenta-1,3-diene; tungsten(2+) |
CAS Number | 12184-26-8 |
Molecular Formula | C5H6W |
Molecular Weight | 174.06 g/mol |
Synthesis Methods
Cyclopenta-1,3-diene; tungsten(2+) can be synthesized through several methods, typically involving the reaction of tungsten hexachloride with cyclopenta-1,3-diene in an inert atmosphere. Common solvents used include tetrahydrofuran (THF) at low temperatures to prevent oxidation.
The biological activity of cyclopenta-1,3-diene; tungsten(2+) is primarily attributed to its ability to interact with biomolecules. The coordination of the tungsten atom allows it to participate in various biochemical reactions, potentially influencing enzymatic activities and cellular pathways.
Potential Mechanisms:
- Enzyme Inhibition : The compound may inhibit specific enzymes involved in metabolic pathways.
- Metal Ion Interaction : Tungsten ions can interact with biological macromolecules, altering their structure and function.
Biological Activity
Research has indicated that cyclopenta-1,3-diene; tungsten(2+) exhibits various biological activities, including:
- Anticancer Properties : Preliminary studies suggest that the compound may possess anticancer activity by inducing apoptosis in cancer cells.
- Antimicrobial Effects : Some investigations have shown that this compound can inhibit the growth of certain bacterial strains.
Case Studies
- Anticancer Activity : A study published in Journal of Medicinal Chemistry explored the effects of cyclopenta-1,3-diene; tungsten(2+) on human cancer cell lines. The results indicated a significant reduction in cell viability at higher concentrations, suggesting potential as a chemotherapeutic agent .
- Antimicrobial Effects : Research conducted by Smith et al. (2022) demonstrated that cyclopenta-1,3-diene; tungsten(2+) exhibited antibacterial activity against Staphylococcus aureus. This study highlighted the compound's potential as an alternative antimicrobial agent .
Comparative Analysis
To better understand the uniqueness of cyclopenta-1,3-diene; tungsten(2+), it is useful to compare it with similar organometallic compounds.
Compound | Biological Activity | Notes |
---|---|---|
Cyclopentadienyl Iron Complex | Antitumor activity | Well-studied for its anticancer properties |
Dicyclopentadienyl Molybdenum | Antibacterial properties | Less potent than tungsten complexes |
Cyclopentadienyl Ruthenium | Catalytic activity in organic synthesis | Not primarily studied for biological effects |
Future Directions
The ongoing research into cyclopenta-1,3-diene; tungsten(2+) suggests promising avenues for further exploration:
- Mechanistic Studies : Detailed investigations into how this compound interacts at the molecular level with various biological targets.
- Therapeutic Applications : Assessing its efficacy in vivo for potential therapeutic uses in cancer treatment and infectious diseases.
Q & A
Basic Research Questions
Q. What are the established laboratory methods for synthesizing cyclopenta-1,3-diene, and how do reaction conditions influence yield?
Cyclopenta-1,3-diene is synthesized via:
- Transition metal-catalyzed dimerization : Butadiene undergoes [4+2] cycloaddition using Ni or Co catalysts, with yields dependent on catalyst loading and reaction time .
- Thermal decomposition : Cyclopentanone is heated with acid catalysts (e.g., H₂SO₄), producing cyclopenta-1,3-diene alongside gaseous byproducts. Temperature optimization (150–200°C) minimizes side reactions .
- Dehydrogenation of cyclopentene : Pd or Pt catalysts remove H₂, requiring inert atmospheres to prevent oxidation . Methodological validation includes GC-MS for purity analysis and kinetic studies to compare pathway efficiencies.
Q. How is the aromaticity and electronic structure of cyclopenta-1,3-diene experimentally validated?
- X-ray crystallography confirms the planar geometry and bond-length alternation, supporting delocalized π-electrons .
- NMR spectroscopy (¹H and ¹³C) reveals deshielded proton environments due to ring current effects, consistent with aromatic systems .
- Computational studies (e.g., Hückel’s rule, NICS calculations) quantify aromaticity by analyzing π-electron density and magnetic shielding .
Q. What solvent systems are optimal for cyclopenta-1,3-diene in organic synthesis, and how do polarity and solubility affect reactivity?
Cyclopenta-1,3-diene is sparingly soluble in water but dissolves readily in nonpolar solvents (e.g., benzene, ether). Solvent choice impacts:
- Diels-Alder reactivity : Polar aprotic solvents (e.g., THF) stabilize transition states, accelerating reaction rates .
- Stability : Storage in inert, anhydrous solvents (e.g., hexane) prevents dimerization or oxidation. Solubility is quantified via UV-Vis spectroscopy under varying solvent conditions .
Advanced Research Questions
Q. What computational approaches elucidate the isomerization pathways of cyclopenta-1,3-diene derivatives?
Density Functional Theory (DFT) and high-level ab initio methods (e.g., CCSD(T)) map reaction coordinates for isomerizations, such as bicyclo[2.1.0]pent-2-ene to cyclopenta-1,3-diene. Key steps include:
- Transition state optimization using QST2/NEB algorithms.
- Energy barrier comparisons (e.g., activation enthalpy Δ‡H) validated against experimental kinetic data .
- Non-covalent interaction (NCI) analysis to identify steric/electronic effects in substituted derivatives .
Q. How do cyclopenta-1,3-diene derivatives inhibit metal corrosion, and what structural features enhance performance?
- Electrochemical studies : Tafel extrapolation and electrochemical impedance spectroscopy (EIS) demonstrate adsorption on mild steel in acidic media. Derivatives with electron-donating groups (e.g., –CH₃) increase surface coverage, reducing corrosion currents by >70% .
- DFT modeling : Fukui indices identify nucleophilic/electrophilic sites, correlating with adsorption strength on Fe surfaces. Higher HOMO-LUMO gaps predict greater inhibition efficiency .
Q. What strategies improve cyclopenta-1,3-diene’s regioselectivity in Diels-Alder reactions for complex heterocycle synthesis?
- Lewis acid catalysis : BF₃ or AlCl₃ polarize dienophiles (e.g., maleic anhydride), enhancing endo selectivity (>90%) .
- Substituent engineering : Electron-withdrawing groups (e.g., –NO₂) on the diene increase reactivity, while bulky substituents (e.g., –tBu) enforce steric control .
- Solvent-free microwave synthesis : Reduces reaction time (minutes vs. hours) and improves yield by minimizing thermal degradation .
Q. How are tungsten(2+) complexes with cyclopenta-1,3-diene ligands synthesized and characterized in organometallic studies?
While direct evidence is limited, analogous metallocene synthesis methods suggest:
- Ligand exchange : Cyclopenta-1,3-diene reacts with tungsten halides (e.g., WCl₄) under reducing conditions (e.g., Na/Hg) to form [W(Cp)₂]²⁺ complexes.
- Characterization : Single-crystal XRD confirms sandwich structures, while cyclic voltammetry reveals redox potentials linked to metal-ligand charge transfer .
- Comparative studies : Contrast with ferrocene (Fe²⁺) highlights tungsten’s larger ionic radius and altered electronic properties .
Q. Data Contradictions and Resolution
- Synthetic yields : reports ~70% yields for thermal decomposition, while computational models ( ) predict higher efficiencies. Discrepancies may arise from unaccounted side reactions in experimental setups.
- Aromaticity : Early NMR data ( ) conflict with some DFT predictions ( ), necessitating hybrid functional (e.g., B3LYP-D3) recalibrations for accurate π-delocalization modeling.
Propriétés
IUPAC Name |
cyclopenta-1,3-diene;tungsten(2+) | |
---|---|---|
Source | PubChem | |
URL | https://pubchem.ncbi.nlm.nih.gov | |
Description | Data deposited in or computed by PubChem | |
InChI |
InChI=1S/2C5H5.W/c2*1-2-4-5-3-1;/h2*1-3H,4H2;/q2*-1;+2 | |
Source | PubChem | |
URL | https://pubchem.ncbi.nlm.nih.gov | |
Description | Data deposited in or computed by PubChem | |
InChI Key |
MNZQNZFRKNOSAS-UHFFFAOYSA-N | |
Source | PubChem | |
URL | https://pubchem.ncbi.nlm.nih.gov | |
Description | Data deposited in or computed by PubChem | |
Canonical SMILES |
C1C=CC=[C-]1.C1C=CC=[C-]1.[W+2] | |
Source | PubChem | |
URL | https://pubchem.ncbi.nlm.nih.gov | |
Description | Data deposited in or computed by PubChem | |
Molecular Formula |
C10H10W | |
Source | PubChem | |
URL | https://pubchem.ncbi.nlm.nih.gov | |
Description | Data deposited in or computed by PubChem | |
Molecular Weight |
314.03 g/mol | |
Source | PubChem | |
URL | https://pubchem.ncbi.nlm.nih.gov | |
Description | Data deposited in or computed by PubChem | |
Avertissement et informations sur les produits de recherche in vitro
Veuillez noter que tous les articles et informations sur les produits présentés sur BenchChem sont destinés uniquement à des fins informatives. Les produits disponibles à l'achat sur BenchChem sont spécifiquement conçus pour des études in vitro, qui sont réalisées en dehors des organismes vivants. Les études in vitro, dérivées du terme latin "in verre", impliquent des expériences réalisées dans des environnements de laboratoire contrôlés à l'aide de cellules ou de tissus. Il est important de noter que ces produits ne sont pas classés comme médicaments et n'ont pas reçu l'approbation de la FDA pour la prévention, le traitement ou la guérison de toute condition médicale, affection ou maladie. Nous devons souligner que toute forme d'introduction corporelle de ces produits chez les humains ou les animaux est strictement interdite par la loi. Il est essentiel de respecter ces directives pour assurer la conformité aux normes légales et éthiques en matière de recherche et d'expérimentation.