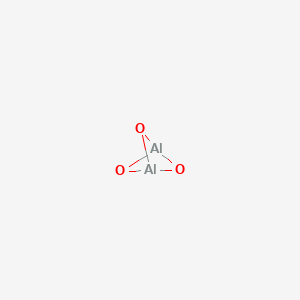
Alumine
Vue d'ensemble
Description
Emery is an odorless, white, crystalline powder. Natural aluminum oxide. Very high melting and hard. Used for polishing, grinding, and abrasive operations. An inhalation hazard when present as a dust.
An oxide of aluminum, occurring in nature as various minerals such as bauxite, corundum, etc. It is used as an adsorbent, desiccating agent, and catalyst, and in the manufacture of dental cements and refractories.
Applications De Recherche Scientifique
Catalyse
Les nanoparticules d'alumine servent de supports de catalyseur efficaces, améliorant les vitesses de réaction et la sélectivité . Leur surface élevée et leur stabilité thermique en font des matériaux idéals pour cette application .
Dispositifs électroniques et optoélectroniques
Les propriétés diélectriques remarquables de l'this compound la rendent précieuse pour les dispositifs électroniques et optoélectroniques . Elle est utilisée dans le domaine de l'électronique comme isolant dans les circuits intégrés et les semi-conducteurs . Elle est également utilisée comme substrat dans la production de dispositifs électroniques à couches minces .
Applications biomédicales
Les nanoparticules d'this compound ont montré des résultats prometteurs dans les applications biomédicales, notamment les systèmes d'administration de médicaments, l'imagerie biomédicale, la biosensibilité et le génie tissulaire . Leur biocompatibilité et leurs capacités de fonctionnalisation de surface les rendent adaptés à ces applications .
Propriétés antimicrobiennes et antivirales
Les nanoparticules d'this compound possèdent de puissantes propriétés antimicrobiennes et antivirales . En raison de leur petite taille, ils peuvent pénétrer plus efficacement les cellules bactériennes et virales, ce qui augmente l'efficacité de leur action . Elles peuvent être incorporées dans des revêtements pour dispositifs médicaux et surfaces hospitalières, contribuant à prévenir l'adhésion bactérienne et la formation de biofilms, réduisant ainsi le risque d'infections
Mécanisme D'action
Target of Action
Alumina, also known as Emery, is a compound that primarily targets various scientific and industrial fields due to its unique bio−/physicochemical properties . These properties include high surface area, high hardness, thermal stability, biocompatibility, surface functionalization, and electrical insulation . In the biomedical field, Alumina nanoparticles have shown promising results in drug delivery systems, biomedical imaging, biosensing, and tissue engineering . Furthermore, they have demonstrated potent antimicrobial and antiviral properties .
Mode of Action
The mode of action of Alumina is primarily physical rather than biochemical. For instance, in the context of its antimicrobial properties, Alumina nanoparticles can penetrate bacterial and viral cells more effectively due to their small size, thereby increasing the efficacy of their action . In the case of insecticide action, charged Alumina particles attach to the insects’ cuticle due to triboelectric forces, absorbing its wax layer by surface area phenomena, resulting in insect dehydration .
Biochemical Pathways
While Alumina primarily acts through physical interactions, it can also influence certain biochemical pathways. For example, in the anaerobic treatment of waste-activated sludge, Alumina slag-modified biochar was found to stimulate the process of short-chain fatty acids (SCFAs) production . This suggests that Alumina can interact with biochemical pathways to enhance certain processes.
Pharmacokinetics
The pharmacokinetics of Alumina, particularly in the context of drug delivery, is an area of active research. One study demonstrated the potential for controlled drug release using a layer-by-layer pH-responsive polyelectrolyte and nanoporous anodic alumina . This suggests that Alumina can be used to control the absorption, distribution, metabolism, and excretion (ADME) of drugs, thereby impacting their bioavailability.
Result of Action
The result of Alumina’s action is multifaceted and depends on its application. In the biomedical field, Alumina nanoparticles can enhance drug delivery, imaging, and sensing capabilities . In the context of antimicrobial properties, Alumina nanoparticles can effectively inhibit bacterial and viral growth . As an insecticide, Alumina can lead to the dehydration and death of insects .
Action Environment
The action, efficacy, and stability of Alumina can be influenced by various environmental factors. For instance, the type of fuel used in Alumina production was found to be a crucial factor in its environmental impact, with potential savings of up to 75.5% of CO2-equivalent emissions . Additionally, the mineral form of bauxite used in Alumina production can also influence its environmental impact . These findings highlight the importance of considering environmental factors in the application and production of Alumina.
Safety and Hazards
Exposure to aluminum is usually not harmful, but exposure to high levels can affect your health . Workers who breathe large amounts of aluminum dusts can have lung problems, such as coughing or abnormal chest X-rays . It is recommended to avoid prolonged or repeated contact with skin, avoid contact with eyes, avoid ingestion and inhalation .
Orientations Futures
Relevant Papers
- “Alumina Ceramic Nanofibers: An Overview of the Spinning Gel Preparation, Manufacturing Process, and Application” provides valuable insights into the design of alumina ceramic nanofibers and explores their potential applications .
- “The effects of energy consumption of alumina production in the…” performs a sensitivity analysis considering possible improvements in efficiency or technological progress .
- “Recent Advances in Nanoporous Anodic Alumina: Principles…” explores the intrinsic properties of alumina porous structures and their vast utility in distinct fields .
Analyse Biochimique
Biochemical Properties
Alumina interacts with various biomolecules in the environment. For instance, in bauxite processing residues, alumina interacts with alkaliphilic bacteria . These bacteria show significant tolerance to metals such as nickel, chromium, aluminum, iron, manganese, magnesium, and lead .
Cellular Effects
The effects of alumina on cells are primarily observed in the context of environmental exposure. Alumina residues, due to their high alkalinity and metal content, can affect the microbial density and activity in the environment .
Molecular Mechanism
It is known that alumina can interact with various enzymes and proteins, potentially influencing their function .
Temporal Effects in Laboratory Settings
In laboratory settings, the effects of alumina can change over time. For instance, the microbial activity in bauxite residues can vary depending on the restoration history of the samples .
Metabolic Pathways
Alumina does not directly participate in metabolic pathways. It can influence these pathways indirectly through its interactions with enzymes and other biomolecules .
Transport and Distribution
Alumina, being a solid compound, does not have a specific transport mechanism within cells or tissues. In the environment, it can be distributed through water and soil .
Subcellular Localization
As a non-biological compound, alumina does not have a specific subcellular localization. When interacting with cells in the environment, it can potentially influence the localization and function of various biomolecules .
Propriétés
IUPAC Name |
oxo(oxoalumanyloxy)alumane | |
---|---|---|
Source | PubChem | |
URL | https://pubchem.ncbi.nlm.nih.gov | |
Description | Data deposited in or computed by PubChem | |
InChI |
InChI=1S/2Al.3O | |
Source | PubChem | |
URL | https://pubchem.ncbi.nlm.nih.gov | |
Description | Data deposited in or computed by PubChem | |
InChI Key |
TWNQGVIAIRXVLR-UHFFFAOYSA-N | |
Source | PubChem | |
URL | https://pubchem.ncbi.nlm.nih.gov | |
Description | Data deposited in or computed by PubChem | |
Canonical SMILES |
O=[Al]O[Al]=O | |
Source | PubChem | |
URL | https://pubchem.ncbi.nlm.nih.gov | |
Description | Data deposited in or computed by PubChem | |
Molecular Formula |
Al2O3 | |
Source | PubChem | |
URL | https://pubchem.ncbi.nlm.nih.gov | |
Description | Data deposited in or computed by PubChem | |
Molecular Weight |
101.961 g/mol | |
Source | PubChem | |
URL | https://pubchem.ncbi.nlm.nih.gov | |
Description | Data deposited in or computed by PubChem | |
Physical Description |
Odorless, white, crystalline powder; [Note: Emery is an impure variety of Al2O3 which may contain small impurities of iron, magnesium & silica. Corundum is natural Al2O3. See also CAS #1302-74-5 (corundum)], Odorless, white, crystalline powder. | |
Record name | Emery | |
Source | Haz-Map, Information on Hazardous Chemicals and Occupational Diseases | |
URL | https://haz-map.com/Agents/478 | |
Description | Haz-Map® is an occupational health database designed for health and safety professionals and for consumers seeking information about the adverse effects of workplace exposures to chemical and biological agents. | |
Explanation | Copyright (c) 2022 Haz-Map(R). All rights reserved. Unless otherwise indicated, all materials from Haz-Map are copyrighted by Haz-Map(R). No part of these materials, either text or image may be used for any purpose other than for personal use. Therefore, reproduction, modification, storage in a retrieval system or retransmission, in any form or by any means, electronic, mechanical or otherwise, for reasons other than personal use, is strictly prohibited without prior written permission. | |
Record name | Emery | |
Source | The National Institute for Occupational Safety and Health (NIOSH) | |
URL | https://www.cdc.gov/niosh/npg/npgd0250.html | |
Description | The NIOSH Pocket Guide to Chemical Hazards is intended as a source of general industrial hygiene information on several hundred chemicals/classes for workers, employers, and occupational health professionals. Read more: https://www.cdc.gov/niosh/npg/ | |
Explanation | The information provided using CDC Web site is only intended to be general summary information to the public. It is not intended to take the place of either the written law or regulations. | |
CAS No. |
1302-74-5, 1317-82-4, 12174-49-1, 12415-34-8 | |
Record name | Corundum | |
Source | ChemIDplus | |
URL | https://pubchem.ncbi.nlm.nih.gov/substance/?source=chemidplus&sourceid=0001302745 | |
Description | ChemIDplus is a free, web search system that provides access to the structure and nomenclature authority files used for the identification of chemical substances cited in National Library of Medicine (NLM) databases, including the TOXNET system. | |
Record name | Sapphire | |
Source | ChemIDplus | |
URL | https://pubchem.ncbi.nlm.nih.gov/substance/?source=chemidplus&sourceid=0001317824 | |
Description | ChemIDplus is a free, web search system that provides access to the structure and nomenclature authority files used for the identification of chemical substances cited in National Library of Medicine (NLM) databases, including the TOXNET system. | |
Record name | Ruby | |
Source | ChemIDplus | |
URL | https://pubchem.ncbi.nlm.nih.gov/substance/?source=chemidplus&sourceid=0012174491 | |
Description | ChemIDplus is a free, web search system that provides access to the structure and nomenclature authority files used for the identification of chemical substances cited in National Library of Medicine (NLM) databases, including the TOXNET system. | |
Record name | Emery | |
Source | ChemIDplus | |
URL | https://pubchem.ncbi.nlm.nih.gov/substance/?source=chemidplus&sourceid=0012415348 | |
Description | ChemIDplus is a free, web search system that provides access to the structure and nomenclature authority files used for the identification of chemical substances cited in National Library of Medicine (NLM) databases, including the TOXNET system. | |
Record name | Corundum (Al2O3) | |
Source | European Chemicals Agency (ECHA) | |
URL | https://echa.europa.eu/information-on-chemicals | |
Description | The European Chemicals Agency (ECHA) is an agency of the European Union which is the driving force among regulatory authorities in implementing the EU's groundbreaking chemicals legislation for the benefit of human health and the environment as well as for innovation and competitiveness. | |
Explanation | Use of the information, documents and data from the ECHA website is subject to the terms and conditions of this Legal Notice, and subject to other binding limitations provided for under applicable law, the information, documents and data made available on the ECHA website may be reproduced, distributed and/or used, totally or in part, for non-commercial purposes provided that ECHA is acknowledged as the source: "Source: European Chemicals Agency, http://echa.europa.eu/". Such acknowledgement must be included in each copy of the material. ECHA permits and encourages organisations and individuals to create links to the ECHA website under the following cumulative conditions: Links can only be made to webpages that provide a link to the Legal Notice page. | |
Record name | Emery | |
Source | European Chemicals Agency (ECHA) | |
URL | https://echa.europa.eu/information-on-chemicals | |
Description | The European Chemicals Agency (ECHA) is an agency of the European Union which is the driving force among regulatory authorities in implementing the EU's groundbreaking chemicals legislation for the benefit of human health and the environment as well as for innovation and competitiveness. | |
Explanation | Use of the information, documents and data from the ECHA website is subject to the terms and conditions of this Legal Notice, and subject to other binding limitations provided for under applicable law, the information, documents and data made available on the ECHA website may be reproduced, distributed and/or used, totally or in part, for non-commercial purposes provided that ECHA is acknowledged as the source: "Source: European Chemicals Agency, http://echa.europa.eu/". Such acknowledgement must be included in each copy of the material. ECHA permits and encourages organisations and individuals to create links to the ECHA website under the following cumulative conditions: Links can only be made to webpages that provide a link to the Legal Notice page. | |
Avertissement et informations sur les produits de recherche in vitro
Veuillez noter que tous les articles et informations sur les produits présentés sur BenchChem sont destinés uniquement à des fins informatives. Les produits disponibles à l'achat sur BenchChem sont spécifiquement conçus pour des études in vitro, qui sont réalisées en dehors des organismes vivants. Les études in vitro, dérivées du terme latin "in verre", impliquent des expériences réalisées dans des environnements de laboratoire contrôlés à l'aide de cellules ou de tissus. Il est important de noter que ces produits ne sont pas classés comme médicaments et n'ont pas reçu l'approbation de la FDA pour la prévention, le traitement ou la guérison de toute condition médicale, affection ou maladie. Nous devons souligner que toute forme d'introduction corporelle de ces produits chez les humains ou les animaux est strictement interdite par la loi. Il est essentiel de respecter ces directives pour assurer la conformité aux normes légales et éthiques en matière de recherche et d'expérimentation.