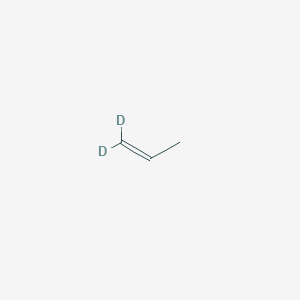
Propene-1,1-D2
Vue d'ensemble
Description
It has the molecular formula C3D2H4 and a molecular weight of 44.09 g/mol . This compound is used in various scientific research applications due to its unique isotopic properties.
Méthodes De Préparation
Synthetic Routes and Reaction Conditions
Propene-1,1-D2 can be synthesized through several methods. One common method involves the dehydrohalogenation of 1,1-dibromo-2-deuteropropane using a strong base such as potassium tert-butoxide . Another method includes the deuteration of propene using deuterium gas in the presence of a catalyst .
Industrial Production Methods
Industrial production of this compound typically involves the catalytic deuteration of propene. This process requires a deuterium source and a suitable catalyst, such as palladium on carbon, to facilitate the exchange of hydrogen atoms with deuterium atoms .
Analyse Des Réactions Chimiques
Types of Reactions
Propene-1,1-D2 undergoes various chemical reactions, including:
Oxidation: This compound can be oxidized to form propene oxide or acrolein under specific conditions.
Reduction: Catalytic hydrogenation of this compound results in the formation of propane-1,1-D2.
Substitution: Halogenation reactions can replace hydrogen atoms with halogen atoms, forming compounds such as 1,1-dibromo-2-deuteropropane.
Common Reagents and Conditions
Oxidation: Common oxidizing agents include potassium permanganate and ozone.
Reduction: Hydrogen gas in the presence of a palladium catalyst is commonly used for hydrogenation reactions.
Substitution: Halogenation reactions often use halogen gases like chlorine or bromine.
Major Products Formed
Oxidation: Propene oxide, acrolein.
Reduction: Propane-1,1-D2.
Substitution: 1,1-dibromo-2-deuteropropane.
Applications De Recherche Scientifique
Scientific Research Applications
Propene-1,1-D2 has several notable applications across different fields of scientific research:
Chemical Kinetics and Mechanisms
- Tracer Studies : this compound is extensively used as a tracer in chemical kinetics studies. The presence of deuterium allows researchers to investigate reaction mechanisms and pathways by observing kinetic isotope effects (KIEs) that arise from the differences in bond strengths between hydrogen and deuterium.
- Isotopic Labeling : The compound serves as an isotopic label in various reactions, enabling the tracking of specific atoms through complex chemical processes .
Biological Studies
- Metabolic Pathways : In biological research, this compound is employed to trace the incorporation of deuterium into biological molecules. This application aids in understanding metabolic processes and mechanisms at a molecular level .
- Drug Development : Deuterated compounds like this compound can enhance the metabolic stability of pharmaceutical compounds. This property is particularly useful in developing new drugs with improved efficacy and reduced toxicity .
Material Science
- Polymer Synthesis : this compound can be utilized in the synthesis of deuterated polymers. These materials often exhibit unique properties that differ from their non-deuterated counterparts, making them valuable for specialized applications in material science.
Several studies have highlighted the applications and benefits of using this compound:
Case Study 1: Kinetic Isotope Effects
A study published in the Journal of Physical Chemistry demonstrated how this compound was used to explore reaction pathways in a complex organic synthesis. The researchers observed significant differences in reaction rates when comparing reactions involving propene versus those involving this compound, thus confirming the utility of deuterated compounds in mechanistic studies.
Case Study 2: Metabolic Tracing
In another study focused on metabolic pathways published in Biochemistry, this compound was employed to trace metabolic incorporation into lipids. The findings indicated that the use of deuterium-labeled substrates could provide insights into lipid metabolism that were previously unattainable with non-deuterated compounds .
Mécanisme D'action
The mechanism of action of propene-1,1-D2 involves the incorporation of deuterium atoms into chemical reactions. Deuterium, being a heavier isotope of hydrogen, affects the reaction kinetics and pathways due to the kinetic isotope effect. This effect results in slower reaction rates for deuterium-labeled compounds compared to their hydrogen counterparts . The molecular targets and pathways involved depend on the specific reaction and application.
Comparaison Avec Des Composés Similaires
Similar Compounds
Propene-1-D1: A mono-deuterated analog of propene with one deuterium atom.
Propene-2-D1: Another mono-deuterated analog with the deuterium atom at a different position.
Propene-D6: A fully deuterated analog with all hydrogen atoms replaced by deuterium.
Uniqueness
Propene-1,1-D2 is unique due to its specific isotopic labeling at the 1,1-positions, which provides distinct kinetic isotope effects and reaction pathways compared to other deuterated analogs . This makes it particularly useful in detailed mechanistic studies and applications requiring precise isotopic labeling.
Activité Biologique
Propene-1,1-D2 (also known as deuterated propene) is a stable isotopic variant of propene where the hydrogen atoms are replaced by deuterium. This modification allows for unique applications in biological and chemical research, particularly in metabolic studies and reaction mechanism investigations. This article explores the biological activity of this compound, focusing on its applications, mechanisms of action, and relevant case studies.
Applications in Biological Research
Metabolic Tracing
this compound is primarily utilized in metabolic studies to trace the incorporation of deuterium into biological molecules. This isotopic labeling helps researchers understand metabolic pathways and the dynamics of biochemical reactions within living organisms.
Development of Deuterated Drugs
In medicinal chemistry, deuterated compounds like this compound are investigated for their potential to improve drug stability and reduce toxicity. Deuteration can enhance the pharmacokinetic properties of drugs, leading to longer half-lives and reduced side effects.
The biological activity of this compound is largely attributed to its isotopic substitution. The presence of deuterium alters bond strengths and reaction kinetics compared to non-deuterated compounds. For instance, in oxidation reactions, the deuterium atom can influence the rate of hydrogen abstraction, leading to different reaction pathways. This phenomenon is particularly relevant in studies involving enzyme kinetics and metabolic pathways .
1. Oxidation Reactions
A study investigated the oxidation of various propenes including this compound over different catalysts. The results indicated that the presence of deuterium significantly affected both the conversion rates and selectivity towards products such as acrolein. For example, under specific conditions (400°C with 15 Torr of propene), the selectivity towards acrolein was markedly influenced by the isotopic composition of the propene used .
Catalyst | Temperature (°C) | Propene Conversion (%) | Acrolein Selectivity (%) |
---|---|---|---|
Sb-Sn Oxide | 400 | 12 | 25 |
SnO2 | 400 | 2.4 | 71 |
2. Metabolic Studies
In metabolic tracing experiments using this compound, researchers have been able to elucidate complex metabolic pathways in various organisms. The incorporation of deuterium into biomolecules allows for precise tracking and quantification of metabolic fluxes. One study demonstrated how deuterated propene could be used to trace lipid metabolism in mammalian cells, revealing insights into fatty acid synthesis and degradation processes .
Research Findings
Recent research highlights several key findings regarding the biological activity of this compound:
- Kinetic Isotope Effects : The substitution of hydrogen with deuterium leads to observable kinetic isotope effects in enzymatic reactions. This can be exploited to infer details about reaction mechanisms and transition states .
- Enhanced Stability : Deuterated compounds often exhibit improved metabolic stability compared to their non-deuterated counterparts. This property is crucial for developing safer pharmaceuticals with prolonged action .
- Biocompatibility : Studies have shown that deuterated compounds can display favorable biocompatibility profiles, making them suitable candidates for therapeutic applications .
Propriétés
IUPAC Name |
1,1-dideuterioprop-1-ene | |
---|---|---|
Source | PubChem | |
URL | https://pubchem.ncbi.nlm.nih.gov | |
Description | Data deposited in or computed by PubChem | |
InChI |
InChI=1S/C3H6/c1-3-2/h3H,1H2,2H3/i1D2 | |
Source | PubChem | |
URL | https://pubchem.ncbi.nlm.nih.gov | |
Description | Data deposited in or computed by PubChem | |
InChI Key |
QQONPFPTGQHPMA-DICFDUPASA-N | |
Source | PubChem | |
URL | https://pubchem.ncbi.nlm.nih.gov | |
Description | Data deposited in or computed by PubChem | |
Canonical SMILES |
CC=C | |
Source | PubChem | |
URL | https://pubchem.ncbi.nlm.nih.gov | |
Description | Data deposited in or computed by PubChem | |
Isomeric SMILES |
[2H]C(=CC)[2H] | |
Source | PubChem | |
URL | https://pubchem.ncbi.nlm.nih.gov | |
Description | Data deposited in or computed by PubChem | |
Molecular Formula |
C3H6 | |
Source | PubChem | |
URL | https://pubchem.ncbi.nlm.nih.gov | |
Description | Data deposited in or computed by PubChem | |
Molecular Weight |
44.09 g/mol | |
Source | PubChem | |
URL | https://pubchem.ncbi.nlm.nih.gov | |
Description | Data deposited in or computed by PubChem | |
Synthesis routes and methods I
Procedure details
Synthesis routes and methods II
Procedure details
Synthesis routes and methods III
Procedure details
Synthesis routes and methods IV
Procedure details
Synthesis routes and methods V
Procedure details
Retrosynthesis Analysis
AI-Powered Synthesis Planning: Our tool employs the Template_relevance Pistachio, Template_relevance Bkms_metabolic, Template_relevance Pistachio_ringbreaker, Template_relevance Reaxys, Template_relevance Reaxys_biocatalysis model, leveraging a vast database of chemical reactions to predict feasible synthetic routes.
One-Step Synthesis Focus: Specifically designed for one-step synthesis, it provides concise and direct routes for your target compounds, streamlining the synthesis process.
Accurate Predictions: Utilizing the extensive PISTACHIO, BKMS_METABOLIC, PISTACHIO_RINGBREAKER, REAXYS, REAXYS_BIOCATALYSIS database, our tool offers high-accuracy predictions, reflecting the latest in chemical research and data.
Strategy Settings
Precursor scoring | Relevance Heuristic |
---|---|
Min. plausibility | 0.01 |
Model | Template_relevance |
Template Set | Pistachio/Bkms_metabolic/Pistachio_ringbreaker/Reaxys/Reaxys_biocatalysis |
Top-N result to add to graph | 6 |
Feasible Synthetic Routes
Q1: Why is propene-1,1-D2 used in studying the reaction between propene and OH radicals?
A1: this compound, along with other deuterated propene isotopes, helps scientists understand the mechanism and rate of hydrogen abstraction by OH radicals. [, ] By substituting specific hydrogen atoms with deuterium, researchers can observe kinetic isotope effects. These effects provide valuable insights into the reaction pathways and which hydrogen atoms are preferentially abstracted by the OH radical.
Q2: What were the key findings regarding the branching ratios of the propene + OH reaction when using this compound?
A2: The research using this compound and other isotopes confirmed that the allylic hydrogen abstraction in propene is the dominant reaction pathway with OH radicals. [, ] This means the hydrogen atom on the carbon adjacent to the double bond is most likely to be abstracted. The studies also provided quantitative data on the rate coefficients for each specific hydrogen abstraction pathway, including the abstraction of the deuterium atoms in this compound, allowing for a comprehensive understanding of the reaction kinetics across a temperature range.
Avertissement et informations sur les produits de recherche in vitro
Veuillez noter que tous les articles et informations sur les produits présentés sur BenchChem sont destinés uniquement à des fins informatives. Les produits disponibles à l'achat sur BenchChem sont spécifiquement conçus pour des études in vitro, qui sont réalisées en dehors des organismes vivants. Les études in vitro, dérivées du terme latin "in verre", impliquent des expériences réalisées dans des environnements de laboratoire contrôlés à l'aide de cellules ou de tissus. Il est important de noter que ces produits ne sont pas classés comme médicaments et n'ont pas reçu l'approbation de la FDA pour la prévention, le traitement ou la guérison de toute condition médicale, affection ou maladie. Nous devons souligner que toute forme d'introduction corporelle de ces produits chez les humains ou les animaux est strictement interdite par la loi. Il est essentiel de respecter ces directives pour assurer la conformité aux normes légales et éthiques en matière de recherche et d'expérimentation.