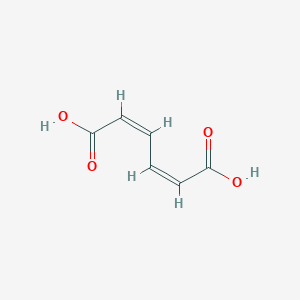
cis,cis-Muconic acid
Vue d'ensemble
Description
L'acide muconique cis,cis est une précieuse plateforme chimique à six atomes de carbone et à deux groupes fonctionnels carboxyliques aux deux extrémités et à deux doubles liaisons au milieu. Il est utilisé comme matière première pour la production de divers polymères et médicaments précieux, notamment l'acide adipique et l'acide téréphtalique
Méthodes De Préparation
Voies de synthèse et conditions de réaction
L'acide muconique cis,cis peut être synthétisé par synthèse chimique à partir du catéchol comme matière première. Cette méthode nécessite des matières premières pétrolières non renouvelables et sensibles à l'environnement, ainsi que de fortes concentrations de catalyseurs métalliques lourds . Le produit final est souvent un mélange de deux isomères : l'acide muconique cis,cis et l'acide muconique cis,trans.
Méthodes de production industrielle
La production industrielle d'acide muconique cis,cis a considérablement progressé avec le développement d'approches biotechnologiques. La production microbienne implique la mise en place de voies de novo dans plusieurs micro-organismes tels qu'Escherichia coli, Corynebacterium glutamicum, Pseudomonas putida et Saccharomyces cerevisiae . Ces méthodes impliquent la refonte des voies métaboliques, le contrôle du flux intermédiaire et l'optimisation du processus de culture pour maximiser le rendement. De plus, la production à partir de la biomasse, telle que la lignine, a attiré l'attention en raison de sa durabilité .
Analyse Des Réactions Chimiques
Types de réactions
L'acide muconique cis,cis subit diverses réactions chimiques, notamment l'isomérisation, l'hydrogénation et la cycloaddition.
Réactifs et conditions courants
Isomérisation : En milieu acide, l'acide muconique cis,cis s'isomérise facilement en son isomère cis,trans.
Cycloaddition : La réaction de Diels-Alder avec de l'éthylène de source biologique peut convertir l'acide muconique cis,cis en acides dicarboxyliques cycliques.
Principaux produits formés
Acide adipique : Un produit important formé par hydrogénation, utilisé dans la production de nylon-6,6.
Acide téréphtalique : Formé par des réactions de cycloaddition, utilisé dans la production de polyéthylène téréphtalate (PET).
Applications de la recherche scientifique
L'acide muconique cis,cis a un large éventail d'applications dans la recherche scientifique :
Chimie : Utilisé comme précurseur pour la synthèse de divers polymères et produits chimiques de spécialité.
Médecine : Applications potentielles dans la synthèse d'intermédiaires pharmaceutiques.
Industrie : Utilisé dans la production de plastiques, de résines et de revêtements bio-sourcés.
Mécanisme d'action
Le mécanisme d'action de l'acide muconique cis,cis implique sa conversion en d'autres produits chimiques précieux par le biais de diverses réactions chimiques. Par exemple, en milieu alcalin, il est déprotoné en l'anion muconate correspondant, qui est stable et ne s'isomérise pas . A l'inverse, en milieu acide, il s'isomérise facilement en son isomère cis,trans . Le processus d'hydrogénation implique des mécanismes de transfert d'électrons couplés aux protons, en particulier sur les nanoparticules de palladium .
Applications De Recherche Scientifique
Overview
Cis,cis-Muconic acid (CCM) is a valuable six-carbon dicarboxylic acid that serves as a versatile platform chemical in various industrial applications, particularly in the production of polymers, pharmaceuticals, and other chemical intermediates. Its significance is underscored by its potential to replace petroleum-derived chemicals, thereby contributing to more sustainable production methods.
Polymer Production
CCM is primarily recognized for its role as a precursor in the synthesis of adipic acid, which is essential for producing nylon-6,6, polyurethanes, and terephthalic acid. The conventional production of adipic acid involves energy-intensive processes that emit greenhouse gases. In contrast, microbial production of CCM represents a more environmentally friendly alternative, utilizing renewable biomass feedstocks.
Application | Description |
---|---|
Nylon Production | Adipic acid derived from CCM is crucial in the manufacture of nylon fibers and plastics. |
Polyurethanes | Used in foams, elastomers, and coatings, providing flexibility and durability. |
Terephthalic Acid | A key ingredient in PET plastics and fibers, enhancing material properties. |
Biotechnological Routes
Recent advances have focused on engineering microorganisms such as Saccharomyces cerevisiae, Escherichia coli, and Corynebacterium glutamicum for the efficient biosynthesis of CCM from renewable resources. This biotechnological approach not only improves yield but also reduces the carbon footprint associated with traditional chemical processes.
- Microbial Production Strategies: Researchers have developed metabolic engineering techniques to enhance CCM production by optimizing pathways such as the shikimate pathway and integrating new biosynthetic routes.
Chemical Intermediates
CCM can be converted into various high-demand chemicals through catalytic processes. For instance:
- Adipic Acid: As mentioned earlier, CCM can be converted into adipic acid.
- Caprolactam: Another important polymer precursor that can be synthesized from CCM.
Case Study 1: Improved Production in Saccharomyces cerevisiae
A study demonstrated the use of a biosensor-aided genome engineering approach to enhance CCM production in S. cerevisiae. By screening mutagenesis libraries, researchers identified genetic targets that significantly increased the yield of CCM during fermentation processes . This method highlights the potential for optimizing microbial strains to meet industrial demands.
Case Study 2: Integrated Process Development
An integrated process for producing CCM from glucose was developed using engineered S. cerevisiae. This study not only focused on maximizing production yields but also established a purification process for large-scale applications. The engineered yeast exhibited robustness in fermentation conditions, making it suitable for industrial scaling .
Future Prospects
The market for this compound is projected to grow significantly due to its applications in sustainable chemistry. With an expected turnover increase from $79.6 million in 2018 to $119.4 million by 2024 , ongoing research into metabolic engineering and bioprocess optimization will likely enhance its commercial viability.
Mécanisme D'action
The mechanism of action of cis,cis-Muconic acid involves its conversion to other valuable chemicals through various chemical reactions. For example, under alkaline conditions, it is deprotonated to the corresponding muconate dianion, which is stable and does not isomerize . Conversely, under acidic conditions, it readily isomerizes to its cis,trans-isomer . The hydrogenation process involves proton-coupled electron transfer mechanisms, particularly on palladium nanoparticles .
Comparaison Avec Des Composés Similaires
L'acide muconique cis,cis est comparé à d'autres acides dicarboxyliques similaires :
Acide adipique : Un produit majeur dérivé de l'acide muconique cis,cis, utilisé dans la production de nylon-6,6.
Acide téréphtalique : Un autre produit dérivé de l'acide muconique cis,cis, utilisé dans la production de PET.
Acide muconique trans,trans : Un isomère de l'acide muconique cis,cis, produit par isomérisation.
L'acide muconique cis,cis est unique en raison de sa capacité à être produit à partir de ressources renouvelables et de sa polyvalence en matière de conversion en divers produits chimiques précieux.
Activité Biologique
cis,cis-Muconic acid (ccMA) is a dicarboxylic acid that serves as a significant platform chemical in the production of various biobased materials, including nylon and other polyamides. Its biological activity is crucial for understanding its potential applications in biotechnology and industrial processes. This article reviews recent research findings, production methods, and biological activities associated with ccMA, highlighting its metabolic pathways, microbial production strategies, and potential applications.
Chemical Structure and Properties
This compound has the molecular formula and features two carboxylic acid groups attached to a six-carbon chain with two double bonds in the cis configuration. This structure imparts unique reactivity characteristics, making it suitable for various chemical transformations.
Metabolic Pathways
ccMA is primarily derived from the shikimate pathway, which is present in many microorganisms. The conversion of aromatic compounds to ccMA involves several enzymatic steps:
- Dehydrogenation of shikimic acid to form 3-dehydroshikimic acid.
- Decarboxylation to protocatechuic acid.
- Cleavage of the aromatic ring to produce ccMA.
Research has shown that various microorganisms can be engineered to enhance ccMA production through modifications in these pathways. For instance, Saccharomyces cerevisiae has been genetically modified to optimize the flux through the shikimate pathway, resulting in increased yields of ccMA .
Microbial Production
Recent advancements in microbial production have focused on optimizing strains such as Escherichia coli, Corynebacterium glutamicum, and Pseudomonas putida. These organisms have been engineered to improve ccMA yields significantly:
- E. coli : Engineered strains achieved yields of up to 4.45 g/L through pathway redesign .
- C. glutamicum : This bacterium has been modified to produce ccMA from lignin-derived aromatic compounds, achieving notable production levels .
- P. putida : Genetic modifications allowed for co-utilization of glucose and xylose, resulting in a titer of 47.2 g/L .
Case Study 1: Engineering Saccharomyces cerevisiae
A study employed a biosensor-based selection method coupled with mutagenesis to enhance ccMA production in S. cerevisiae. The engineered strain produced 20.8 g/L of ccMA during fed-batch fermentation, representing a significant increase compared to previous metrics . This study highlights the potential of synthetic biology approaches in optimizing microbial production systems.
Case Study 2: Lignin Valorization
Research by Becher et al. demonstrated the conversion of lignin-derived aromatic compounds into ccMA using engineered C. glutamicum. This approach not only provides an alternative feedstock but also contributes to sustainable practices by utilizing waste materials . The study reported successful hydrothermal treatment of lignin, leading to efficient conversion pathways.
Applications
ccMA's versatility extends beyond its role as a precursor for nylon and polyurethanes; it is also utilized in producing biodegradable plastics and as an intermediate for various pharmaceuticals. Its dual functionality as a building block for polymers makes it an attractive candidate for sustainable material science.
Data Summary
Propriétés
IUPAC Name |
(2Z,4Z)-hexa-2,4-dienedioic acid | |
---|---|---|
Source | PubChem | |
URL | https://pubchem.ncbi.nlm.nih.gov | |
Description | Data deposited in or computed by PubChem | |
InChI |
InChI=1S/C6H6O4/c7-5(8)3-1-2-4-6(9)10/h1-4H,(H,7,8)(H,9,10)/b3-1-,4-2- | |
Source | PubChem | |
URL | https://pubchem.ncbi.nlm.nih.gov | |
Description | Data deposited in or computed by PubChem | |
InChI Key |
TXXHDPDFNKHHGW-CCAGOZQPSA-N | |
Source | PubChem | |
URL | https://pubchem.ncbi.nlm.nih.gov | |
Description | Data deposited in or computed by PubChem | |
Canonical SMILES |
C(=CC(=O)O)C=CC(=O)O | |
Source | PubChem | |
URL | https://pubchem.ncbi.nlm.nih.gov | |
Description | Data deposited in or computed by PubChem | |
Isomeric SMILES |
C(=C\C(=O)O)\C=C/C(=O)O | |
Source | PubChem | |
URL | https://pubchem.ncbi.nlm.nih.gov | |
Description | Data deposited in or computed by PubChem | |
Molecular Formula |
C6H6O4 | |
Source | PubChem | |
URL | https://pubchem.ncbi.nlm.nih.gov | |
Description | Data deposited in or computed by PubChem | |
DSSTOX Substance ID |
DTXSID801315323 | |
Record name | cis,cis-Muconic acid | |
Source | EPA DSSTox | |
URL | https://comptox.epa.gov/dashboard/DTXSID801315323 | |
Description | DSSTox provides a high quality public chemistry resource for supporting improved predictive toxicology. | |
Molecular Weight |
142.11 g/mol | |
Source | PubChem | |
URL | https://pubchem.ncbi.nlm.nih.gov | |
Description | Data deposited in or computed by PubChem | |
Physical Description |
Solid | |
Record name | cis,cis-Muconic acid | |
Source | Human Metabolome Database (HMDB) | |
URL | http://www.hmdb.ca/metabolites/HMDB0006331 | |
Description | The Human Metabolome Database (HMDB) is a freely available electronic database containing detailed information about small molecule metabolites found in the human body. | |
Explanation | HMDB is offered to the public as a freely available resource. Use and re-distribution of the data, in whole or in part, for commercial purposes requires explicit permission of the authors and explicit acknowledgment of the source material (HMDB) and the original publication (see the HMDB citing page). We ask that users who download significant portions of the database cite the HMDB paper in any resulting publications. | |
CAS No. |
1119-72-8 | |
Record name | cis,cis-Muconic acid | |
Source | CAS Common Chemistry | |
URL | https://commonchemistry.cas.org/detail?cas_rn=1119-72-8 | |
Description | CAS Common Chemistry is an open community resource for accessing chemical information. Nearly 500,000 chemical substances from CAS REGISTRY cover areas of community interest, including common and frequently regulated chemicals, and those relevant to high school and undergraduate chemistry classes. This chemical information, curated by our expert scientists, is provided in alignment with our mission as a division of the American Chemical Society. | |
Explanation | The data from CAS Common Chemistry is provided under a CC-BY-NC 4.0 license, unless otherwise stated. | |
Record name | cis,cis-Muconic acid | |
Source | ChemIDplus | |
URL | https://pubchem.ncbi.nlm.nih.gov/substance/?source=chemidplus&sourceid=0001119728 | |
Description | ChemIDplus is a free, web search system that provides access to the structure and nomenclature authority files used for the identification of chemical substances cited in National Library of Medicine (NLM) databases, including the TOXNET system. | |
Record name | cis,cis-Muconic acid | |
Source | EPA DSSTox | |
URL | https://comptox.epa.gov/dashboard/DTXSID801315323 | |
Description | DSSTox provides a high quality public chemistry resource for supporting improved predictive toxicology. | |
Record name | cis,cis-Muconic acid | |
Source | European Chemicals Agency (ECHA) | |
URL | https://echa.europa.eu/information-on-chemicals | |
Description | The European Chemicals Agency (ECHA) is an agency of the European Union which is the driving force among regulatory authorities in implementing the EU's groundbreaking chemicals legislation for the benefit of human health and the environment as well as for innovation and competitiveness. | |
Explanation | Use of the information, documents and data from the ECHA website is subject to the terms and conditions of this Legal Notice, and subject to other binding limitations provided for under applicable law, the information, documents and data made available on the ECHA website may be reproduced, distributed and/or used, totally or in part, for non-commercial purposes provided that ECHA is acknowledged as the source: "Source: European Chemicals Agency, http://echa.europa.eu/". Such acknowledgement must be included in each copy of the material. ECHA permits and encourages organisations and individuals to create links to the ECHA website under the following cumulative conditions: Links can only be made to webpages that provide a link to the Legal Notice page. | |
Record name | MUCONIC ACID, (Z,Z)- | |
Source | FDA Global Substance Registration System (GSRS) | |
URL | https://gsrs.ncats.nih.gov/ginas/app/beta/substances/2G78TUQ51G | |
Description | The FDA Global Substance Registration System (GSRS) enables the efficient and accurate exchange of information on what substances are in regulated products. Instead of relying on names, which vary across regulatory domains, countries, and regions, the GSRS knowledge base makes it possible for substances to be defined by standardized, scientific descriptions. | |
Explanation | Unless otherwise noted, the contents of the FDA website (www.fda.gov), both text and graphics, are not copyrighted. They are in the public domain and may be republished, reprinted and otherwise used freely by anyone without the need to obtain permission from FDA. Credit to the U.S. Food and Drug Administration as the source is appreciated but not required. | |
Record name | cis,cis-Muconic acid | |
Source | Human Metabolome Database (HMDB) | |
URL | http://www.hmdb.ca/metabolites/HMDB0006331 | |
Description | The Human Metabolome Database (HMDB) is a freely available electronic database containing detailed information about small molecule metabolites found in the human body. | |
Explanation | HMDB is offered to the public as a freely available resource. Use and re-distribution of the data, in whole or in part, for commercial purposes requires explicit permission of the authors and explicit acknowledgment of the source material (HMDB) and the original publication (see the HMDB citing page). We ask that users who download significant portions of the database cite the HMDB paper in any resulting publications. | |
Retrosynthesis Analysis
AI-Powered Synthesis Planning: Our tool employs the Template_relevance Pistachio, Template_relevance Bkms_metabolic, Template_relevance Pistachio_ringbreaker, Template_relevance Reaxys, Template_relevance Reaxys_biocatalysis model, leveraging a vast database of chemical reactions to predict feasible synthetic routes.
One-Step Synthesis Focus: Specifically designed for one-step synthesis, it provides concise and direct routes for your target compounds, streamlining the synthesis process.
Accurate Predictions: Utilizing the extensive PISTACHIO, BKMS_METABOLIC, PISTACHIO_RINGBREAKER, REAXYS, REAXYS_BIOCATALYSIS database, our tool offers high-accuracy predictions, reflecting the latest in chemical research and data.
Strategy Settings
Precursor scoring | Relevance Heuristic |
---|---|
Min. plausibility | 0.01 |
Model | Template_relevance |
Template Set | Pistachio/Bkms_metabolic/Pistachio_ringbreaker/Reaxys/Reaxys_biocatalysis |
Top-N result to add to graph | 6 |
Feasible Synthetic Routes
Q1: What is the significance of microbial production of cis,cis-muconic acid?
A1: Microbial production of ccMA offers a sustainable alternative to petroleum-based methods, contributing to a greener chemical industry. [, , ]
Q2: How is this compound produced from renewable feedstocks?
A2: ccMA can be synthesized from biomass, primarily through the microbial conversion of sugars and lignin-derived aromatic compounds. Engineered microorganisms, such as Pseudomonas putida and Saccharomyces cerevisiae, are employed for this purpose. [, , , ]
Q3: What are the key metabolic pathways involved in the biosynthesis of this compound?
A3: Several pathways lead to ccMA biosynthesis. The β-ketoadipate pathway, involving the ortho-cleavage of catechol, is commonly utilized. The L-lysine pathway and the reverse β-oxidation pathway represent alternative routes. [, , , ]
Q4: What are the challenges associated with microbial production of this compound?
A4: Low production titers, intermediate accumulation, and competition with cell growth for resources are major challenges. Optimization strategies include engineering metabolic pathways, controlling intermediate flux, and strain optimization. [, , , ]
Q5: Can this compound be produced from lignin without using glucose?
A5: Yes. Researchers have engineered Pseudomonas putida and Sphingobium sp. strains capable of producing ccMA from lignin model compounds without glucose supplementation. This achievement is crucial for utilizing both softwood and hardwood lignin for ccMA production. []
Q6: What is the molecular formula, weight, and spectroscopic data of this compound?
A6: The molecular formula of ccMA is C6H6O4. It has a molecular weight of 142.11 g/mol. Spectroscopic data, including 1H NMR and 13C NMR, can confirm its cis,cis-configuration and distinguish it from other isomers. [, , ]
Q7: What is the importance of the cis,cis-configuration in muconic acid for further applications?
A7: The cis,cis-configuration is crucial for the Diels-Alder reaction, which enables the synthesis of various cyclic compounds from ccMA. []
Q8: How does the pH of the solution influence the reactivity of this compound?
A8: The reactivity of ccMA is highly pH-dependent. Under alkaline conditions, ccMA exists as a stable dianion that resists isomerization. In acidic environments, it readily isomerizes to its cis,trans-form. Further heating under acidic conditions promotes intramolecular cyclization to form lactones. []
Q9: What strategies can be employed to favor the selective isomerization of this compound to trans,trans-muconic acid?
A9: Chelation of carboxylate groups with inorganic salts or solvation using polar aprotic solvents hinders ring-closing reactions, thereby promoting the selective isomerization to trans,trans-muconic acid. []
Q10: What is the significance of this compound as a platform chemical?
A10: ccMA serves as a versatile precursor for various valuable chemicals. Its most notable application is its conversion to adipic acid, a key monomer in nylon production. It also serves as a precursor for terephthalic acid, used in polyethylene terephthalate (PET) synthesis. [, , , ]
Q11: How is this compound converted to adipic acid?
A11: ccMA is catalytically hydrogenated to produce adipic acid. Various catalysts, including platinum group metals such as palladium and rhodium supported on activated carbon or silica, have shown promising activity and selectivity. []
Q12: What are the challenges associated with the catalytic conversion of biologically derived muconic acid to adipic acid?
A12: Achieving the high purity of adipic acid (99.8%) required for nylon synthesis necessitates efficient separation and purification processes. Catalyst stability and leaching of metals also pose challenges. []
Q13: Beyond adipic acid, what other valuable chemicals can be derived from this compound?
A13: ccMA can be converted to other valuable compounds like terephthalic acid, 1,4-cyclohexanedicarboxylic acid, and various biobased cyclic C6-1,4-diacid monomers. These compounds are essential building blocks for polymers and other performance materials. [, ]
Q14: What are the environmental benefits of using this compound as a platform chemical?
A14: Utilizing ccMA derived from renewable resources contributes to a more sustainable chemical industry by reducing reliance on petroleum-based feedstocks and promoting a circular economy. [, , ]
Q15: What are the potential environmental impacts of this compound production and its use?
A15: While considered more environmentally friendly than petroleum-based methods, the environmental impact of ccMA production should be carefully evaluated. Factors to consider include land use for biomass cultivation, energy consumption during fermentation and downstream processing, and the potential for water pollution. []
Q16: What strategies can be implemented to mitigate the environmental impact of this compound production?
A16: Minimizing waste generation, employing energy-efficient processes, and developing closed-loop systems for water and solvent recycling are crucial for mitigating the environmental footprint of ccMA production. [, ]
Q17: What are the current research frontiers in this compound production?
A17: Current research focuses on improving microbial strain performance for enhanced ccMA titers, yields, and productivities. Exploring new metabolic pathways, developing efficient and cost-effective separation and purification technologies, and optimizing downstream catalytic conversion processes are key areas of ongoing research. [, , ]
Q18: What are the future prospects and challenges for the industrial-scale production of this compound?
A18: Scaling up ccMA production to meet industrial demands requires overcoming challenges related to process economics, strain stability, and efficient downstream processing. Collaboration between academia and industry is crucial for translating laboratory-scale successes into commercially viable processes. [, ]
Q19: What are the potential societal benefits of transitioning to a bio-based economy using platform chemicals like this compound?
A19: A bio-based economy reliant on renewable resources like ccMA has the potential to reduce greenhouse gas emissions, decrease dependence on fossil fuels, create new job opportunities in rural communities, and promote sustainable economic growth. []
Avertissement et informations sur les produits de recherche in vitro
Veuillez noter que tous les articles et informations sur les produits présentés sur BenchChem sont destinés uniquement à des fins informatives. Les produits disponibles à l'achat sur BenchChem sont spécifiquement conçus pour des études in vitro, qui sont réalisées en dehors des organismes vivants. Les études in vitro, dérivées du terme latin "in verre", impliquent des expériences réalisées dans des environnements de laboratoire contrôlés à l'aide de cellules ou de tissus. Il est important de noter que ces produits ne sont pas classés comme médicaments et n'ont pas reçu l'approbation de la FDA pour la prévention, le traitement ou la guérison de toute condition médicale, affection ou maladie. Nous devons souligner que toute forme d'introduction corporelle de ces produits chez les humains ou les animaux est strictement interdite par la loi. Il est essentiel de respecter ces directives pour assurer la conformité aux normes légales et éthiques en matière de recherche et d'expérimentation.