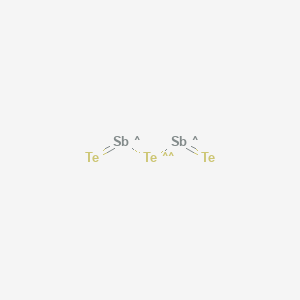
Tellure d'antimoine(III)
- Cliquez sur DEMANDE RAPIDE pour recevoir un devis de notre équipe d'experts.
- Avec des produits de qualité à un prix COMPÉTITIF, vous pouvez vous concentrer davantage sur votre recherche.
Vue d'ensemble
Description
Antimony(III) telluride is an inorganic compound with the chemical formula Sb₂Te₃. It is a grey crystalline solid with a layered structure, consisting of two atomic sheets of antimony and three atomic sheets of tellurium held together by weak van der Waals forces . This compound is a narrow-gap semiconductor with a band gap of 0.21 eV and is also known for its topological insulator properties .
Applications De Recherche Scientifique
Antimony(III) telluride has a wide range of applications in scientific research:
Thermoelectric Materials: It is used in the fabrication of thermoelectric materials due to its large thermoelectric effect.
Semiconductors: It can be transformed into both n-type and p-type semiconductors by doping with appropriate dopants.
Phase-Change Memory Devices: Its reversible phase-change behavior makes it suitable for non-volatile random access memory applications.
Solid State Refrigerators: Due to its thermoelectric properties, it is used in solid state refrigerators.
Mécanisme D'action
Target of Action
Antimony(III) telluride (Sb2Te3) is a three-dimensional topological insulator . It primarily targets the formation of thermoelectric materials, where it forms p-type semiconducting films .
Mode of Action
Antimony(III) telluride interacts with its targets by forming a layered structure. These layers consist of two atomic sheets of antimony and three atomic sheets of tellurium, held together by weak van der Waals forces . This compound can be transformed into both n-type and p-type semiconductors when doped with an appropriate dopant .
Biochemical Pathways
It’s known that doping sb2te3 with iron introduces multiple fermi pockets, resulting in reduced carrier density and mobility .
Pharmacokinetics
It’s known that due to its layered structure and weak inter-layer forces, bulk antimony telluride may be mechanically exfoliated to isolate single sheets .
Result of Action
The primary result of Antimony(III) telluride’s action is the formation of thermoelectric materials . It also forms the pseudobinary intermetallic system germanium-antimony-telluride with germanium telluride .
Action Environment
Environmental factors can influence the action, efficacy, and stability of Antimony(III) telluride. For instance, it’s known that the compound’s properties exhibit thickness-dependence . .
Analyse Biochimique
Biochemical Properties
Antimony(III) telluride has been investigated for its semiconductor properties . It can be transformed into both n-type and p-type semiconductors by doping with an appropriate dopant . There is currently limited information available on the specific enzymes, proteins, and other biomolecules that Antimony(III) telluride interacts with in biochemical reactions.
Molecular Mechanism
It is known that doping Antimony(III) telluride with iron introduces multiple Fermi pockets, in contrast to the single frequency detected for pure Antimony(III) telluride, and results in reduced carrier density and mobility . The specifics of how Antimony(III) telluride exerts its effects at the molecular level,
Méthodes De Préparation
Synthetic Routes and Reaction Conditions: Antimony(III) telluride can be synthesized by reacting antimony with tellurium at high temperatures ranging from 500°C to 900°C . The reaction is as follows: [ 2 \text{Sb} (l) + 3 \text{Te} (l) \rightarrow \text{Sb}_2\text{Te}_3 (l) ]
Industrial Production Methods: In industrial settings, antimony(III) telluride is often produced through high-pressure synthesis, ball milling, and spark plasma sintering. These methods help in achieving high purity and desired microstructures .
Analyse Des Réactions Chimiques
Types of Reactions:
Oxidation: Antimony(III) telluride can undergo oxidation to form antimony(V) telluride.
Reduction: It can be reduced back to its elemental forms under certain conditions.
Substitution: It can participate in substitution reactions where tellurium atoms are replaced by other chalcogens like sulfur or selenium.
Common Reagents and Conditions:
Oxidizing Agents: Hydrogen peroxide can oxidize antimony(III) telluride under alkaline conditions.
Reducing Agents: Hydrazine vapor can be used for reduction processes.
Substitution Reactions: Reactions with sulfur or selenium at elevated temperatures can lead to the formation of antimony(III) sulfide or antimony(III) selenide.
Major Products:
Oxidation: Antimony(V) telluride.
Reduction: Elemental antimony and tellurium.
Substitution: Antimony(III) sulfide or antimony(III) selenide.
Comparaison Avec Des Composés Similaires
Bismuth(III) telluride (Bi₂Te₃): Similar in structure and thermoelectric properties.
Germanium telluride (GeTe): Forms pseudobinary intermetallic systems with antimony(III) telluride.
Antimony(III) selenide (Sb₂Se₃): Another chalcogenide with similar properties.
Uniqueness: Antimony(III) telluride is unique due to its topological insulator properties, which are not commonly found in other similar compounds. This property allows it to exhibit thickness-dependent physical properties, making it highly versatile for various applications .
Propriétés
Numéro CAS |
1327-50-0 |
---|---|
Formule moléculaire |
SbTe |
Poids moléculaire |
249.4 g/mol |
Nom IUPAC |
antimony;tellurium |
InChI |
InChI=1S/Sb.Te |
Clé InChI |
DDJAGKOCVFYQOV-UHFFFAOYSA-N |
SMILES |
[Sb]=[Te].[Sb]=[Te].[Te] |
SMILES canonique |
[Sb].[Te] |
Origine du produit |
United States |
Q1: What are the promising applications of Antimony (III) telluride in laser technology?
A1: Antimony (III) telluride exhibits nonlinear optical properties, making it suitable as a saturable absorber in Q-switched Erbium-doped fiber lasers (EDFLs). Research has shown that incorporating Sb2Te3-PVA thin films into EDFL cavities enables the generation of stable and self-starting Q-switched pulses at a center wavelength of 1560 nm. [, ] These lasers demonstrate desirable characteristics such as short pulse widths, high repetition rates, and high signal-to-noise ratios, making them suitable for applications in optical communication, sensing, and material processing. [, ]
Q2: How does the incorporation of Antimony (III) telluride affect the thermoelectric properties of materials?
A2: Antimony (III) telluride is a known thermoelectric material. Studies have explored the incorporation of Platinum (Pt) nanocrystals into Sb2Te3 thin films to form nanocomposites with enhanced thermoelectric properties. [] The introduction of Pt nanocrystals facilitates carrier energy filtering by scattering low-energy holes, leading to an increase in thermopower. [] Although the presence of Pt nanocrystals increases carrier concentration, it partially offsets the reduction in electrical conductivity caused by decreased mobility. [] This approach demonstrates a promising strategy for improving the thermoelectric performance of Sb2Te3-based materials for applications in waste heat recovery and solid-state cooling.
Q3: How is Antimony (III) telluride utilized in thin-film cooling devices?
A3: Antimony (III) telluride plays a crucial role in multilayer thin-film cooling devices. Research has demonstrated the fabrication of in-plane solid-state thermoelectric cooling devices using multilayered Bi2Te3/Sb2Te3 thin films. [] These devices leverage the Peltier effect, where electricity is used to pump heat from the cold end to the hot end. [] The fabrication process involves depositing alternating layers of Bi2Te3 and Sb2Te3 using e-beam evaporation, creating a periodic structure that enhances thermoelectric properties. [] These devices hold promise for applications requiring highly efficient micro-cooling, such as thermal management in electronic devices and integrated circuits.
Q4: What is the structural characterization of Antimony (III) telluride?
A4: Antimony (III) telluride is a chemical compound with the formula Sb2Te3. It possesses a rhombohedral crystal structure and belongs to the space group R3̅m. Its molecular weight is 626.32 g/mol. While specific spectroscopic data wasn't provided in the research excerpts, common characterization techniques for Sb2Te3 include X-ray diffraction (XRD) for crystal structure analysis, scanning electron microscopy (SEM) for surface morphology, and energy-dispersive X-ray spectroscopy (EDX) for elemental composition analysis.
Q5: How does Antimony (III) telluride compare to other chalcogenides in Atomic Layer Deposition (ALD) processes?
A5: Antimony (III) telluride, similar to other layered chalcogenides, presents challenges in ALD due to the van der Waals bonding between layers. [] Research indicates that achieving a continuous Sb2Te3 layer via ALD is highly dependent on the starting surface and requires a significant number of deposition cycles. [] For instance, deposition on the van der Waals surface of materials like MoS2 proves difficult. [] This highlights the need for further research to optimize ALD processes for Sb2Te3 and similar materials to achieve controlled and uniform thin film growth.
Avertissement et informations sur les produits de recherche in vitro
Veuillez noter que tous les articles et informations sur les produits présentés sur BenchChem sont destinés uniquement à des fins informatives. Les produits disponibles à l'achat sur BenchChem sont spécifiquement conçus pour des études in vitro, qui sont réalisées en dehors des organismes vivants. Les études in vitro, dérivées du terme latin "in verre", impliquent des expériences réalisées dans des environnements de laboratoire contrôlés à l'aide de cellules ou de tissus. Il est important de noter que ces produits ne sont pas classés comme médicaments et n'ont pas reçu l'approbation de la FDA pour la prévention, le traitement ou la guérison de toute condition médicale, affection ou maladie. Nous devons souligner que toute forme d'introduction corporelle de ces produits chez les humains ou les animaux est strictement interdite par la loi. Il est essentiel de respecter ces directives pour assurer la conformité aux normes légales et éthiques en matière de recherche et d'expérimentation.