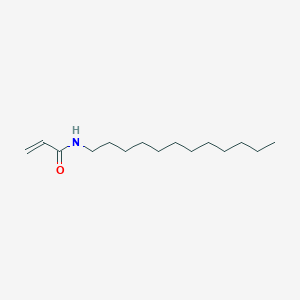
N-Dodécylacrylamide
Vue d'ensemble
Description
N-Dodecylacrylamide is an organic compound with the molecular formula C15H29NO. It is a derivative of acrylamide, where the hydrogen atom of the amide group is replaced by a dodecyl group. This compound is known for its unique properties, such as forming highly ordered lamellar structures when polymerized . It is used in various scientific and industrial applications due to its amphiphilic nature, which allows it to interact with both hydrophobic and hydrophilic substances.
Applications De Recherche Scientifique
N-Dodecylacrylamide has a wide range of applications in scientific research:
Chemistry: It is used in the synthesis of polymers and copolymers with unique structural properties.
Biology: Its amphiphilic nature makes it useful in the study of cell membranes and other biological interfaces.
Medicine: It is explored for use in drug delivery systems due to its ability to form micelles and other nanostructures.
Mécanisme D'action
Target of Action
N-Dodecylacrylamide (pDDA) is a unique compound that primarily targets the formation of highly ordered lamellar structures . The compound’s primary role is to induce the formation of these structures, which are crucial in various applications, particularly in the field of polymer science .
Mode of Action
The compound interacts with its targets by inducing the formation of well-ordered lamellar structures through a process known as humid annealing . This process involves the crystallized part of the dodecyl side chain in nanodomains melting at −28.5 °C and a glass transition temperature of 73.6 °C . This interaction results in changes in the physical properties of the compound, making it an amorphous polymer at room temperature (20 °C) .
Biochemical Pathways
The primary biochemical pathway affected by N-Dodecylacrylamide is the formation of lamellar structures. The compound induces the formation of these structures by interacting with the dodecyl side chains and main chains, which contain water-adsorbed amide groups . The segregation between these chains is the driving force for the observed structural changes .
Pharmacokinetics
It’s known that the compound’s properties, such as its low solubility and high viscosity , can impact its bioavailability.
Result of Action
The result of N-Dodecylacrylamide’s action is the formation of well-ordered lamellar structures. These structures are formed when the compound is annealed under 98% relative humidity at 60 °C for 24 hours . The lamellar spacing is determined to be 3.25 nm from the Bragg peak . Fourier transform infrared measurements of lamellar structured films show that the dodecyl side chains are aligned perpendicular to the lamellar plane .
Action Environment
The action of N-Dodecylacrylamide is influenced by environmental factors such as temperature and humidity. For instance, the compound forms well-ordered lamellar structures when annealed under 98% relative humidity at 60 °C . This suggests that the compound’s action, efficacy, and stability are highly dependent on the environmental conditions.
Safety and Hazards
Analyse Biochimique
Biochemical Properties
N-Dodecylacrylamide, when subjected to humid annealing, forms a well-ordered lamellar structure . This structure is composed of randomly oriented lamellar domains . The lamellar spacing was determined to be 3.25 nm from the Bragg peak .
Cellular Effects
The formation of its well-ordered lamellar structure suggests potential interactions with cellular components .
Molecular Mechanism
The molecular mechanism of N-Dodecylacrylamide involves the formation of a well-ordered lamellar structure by annealing in humid conditions . The dodecyl side chains are aligned perpendicular to the lamellar plane .
Temporal Effects in Laboratory Settings
In laboratory settings, N-Dodecylacrylamide exhibits changes over time. For instance, when the compound was annealed under 98% relative humidity at 60 °C for 24 hours, the lamellar plane oriented parallel to the substrate plane .
Méthodes De Préparation
Synthetic Routes and Reaction Conditions: N-Dodecylacrylamide is synthesized by reacting N-dodecylamine with acryloyl chloride in the presence of triethylamine. The reaction typically takes place in an organic solvent such as 1,2-dichloroethane. The product is then purified by column chromatography and recrystallization from n-hexane .
Industrial Production Methods: In industrial settings, the synthesis of N-Dodecylacrylamide follows similar routes but on a larger scale. The reaction conditions are optimized to ensure high yield and purity. The use of continuous flow reactors and automated purification systems enhances the efficiency of the production process.
Analyse Des Réactions Chimiques
Types of Reactions: N-Dodecylacrylamide undergoes various chemical reactions, including:
Polymerization: It can polymerize to form poly(N-dodecylacrylamide), which exhibits unique structural properties.
Copolymerization: It can copolymerize with other monomers such as methyl methacrylate to form copolymers with tailored properties.
Common Reagents and Conditions:
Polymerization: Free radical initiators such as 2,2′-azobis(isobutyronitrile) are commonly used in the polymerization process.
Copolymerization: Similar conditions are used for copolymerization, with the addition of the comonomer and appropriate initiators.
Major Products:
Poly(N-dodecylacrylamide): A polymer with highly ordered lamellar structures.
Copolymers: Depending on the comonomers used, various copolymers with different properties can be synthesized.
Comparaison Avec Des Composés Similaires
N-Octylacrylamide: Similar to N-Dodecylacrylamide but with a shorter alkyl chain.
N-Octadecylacrylamide: Has a longer alkyl chain and forms more stable structures but with different properties compared to N-Dodecylacrylamide.
Uniqueness: N-Dodecylacrylamide is unique due to its ability to form highly ordered lamellar structures, which are not as easily formed by its shorter or longer chain analogs . This property makes it particularly useful in applications requiring precise structural organization.
Propriétés
IUPAC Name |
N-dodecylprop-2-enamide | |
---|---|---|
Source | PubChem | |
URL | https://pubchem.ncbi.nlm.nih.gov | |
Description | Data deposited in or computed by PubChem | |
InChI |
InChI=1S/C15H29NO/c1-3-5-6-7-8-9-10-11-12-13-14-16-15(17)4-2/h4H,2-3,5-14H2,1H3,(H,16,17) | |
Source | PubChem | |
URL | https://pubchem.ncbi.nlm.nih.gov | |
Description | Data deposited in or computed by PubChem | |
InChI Key |
XQPVIMDDIXCFFS-UHFFFAOYSA-N | |
Source | PubChem | |
URL | https://pubchem.ncbi.nlm.nih.gov | |
Description | Data deposited in or computed by PubChem | |
Canonical SMILES |
CCCCCCCCCCCCNC(=O)C=C | |
Source | PubChem | |
URL | https://pubchem.ncbi.nlm.nih.gov | |
Description | Data deposited in or computed by PubChem | |
Molecular Formula |
C15H29NO | |
Source | PubChem | |
URL | https://pubchem.ncbi.nlm.nih.gov | |
Description | Data deposited in or computed by PubChem | |
Related CAS |
33827-81-5 | |
Record name | 2-Propenamide, N-dodecyl-, homopolymer | |
Source | CAS Common Chemistry | |
URL | https://commonchemistry.cas.org/detail?cas_rn=33827-81-5 | |
Description | CAS Common Chemistry is an open community resource for accessing chemical information. Nearly 500,000 chemical substances from CAS REGISTRY cover areas of community interest, including common and frequently regulated chemicals, and those relevant to high school and undergraduate chemistry classes. This chemical information, curated by our expert scientists, is provided in alignment with our mission as a division of the American Chemical Society. | |
Explanation | The data from CAS Common Chemistry is provided under a CC-BY-NC 4.0 license, unless otherwise stated. | |
DSSTOX Substance ID |
DTXSID50164580 | |
Record name | N-Dodecylacrylamide | |
Source | EPA DSSTox | |
URL | https://comptox.epa.gov/dashboard/DTXSID50164580 | |
Description | DSSTox provides a high quality public chemistry resource for supporting improved predictive toxicology. | |
Molecular Weight |
239.40 g/mol | |
Source | PubChem | |
URL | https://pubchem.ncbi.nlm.nih.gov | |
Description | Data deposited in or computed by PubChem | |
CAS No. |
1506-53-2 | |
Record name | N-Dodecyl-2-propenamide | |
Source | CAS Common Chemistry | |
URL | https://commonchemistry.cas.org/detail?cas_rn=1506-53-2 | |
Description | CAS Common Chemistry is an open community resource for accessing chemical information. Nearly 500,000 chemical substances from CAS REGISTRY cover areas of community interest, including common and frequently regulated chemicals, and those relevant to high school and undergraduate chemistry classes. This chemical information, curated by our expert scientists, is provided in alignment with our mission as a division of the American Chemical Society. | |
Explanation | The data from CAS Common Chemistry is provided under a CC-BY-NC 4.0 license, unless otherwise stated. | |
Record name | N-Dodecylacrylamide | |
Source | ChemIDplus | |
URL | https://pubchem.ncbi.nlm.nih.gov/substance/?source=chemidplus&sourceid=0001506532 | |
Description | ChemIDplus is a free, web search system that provides access to the structure and nomenclature authority files used for the identification of chemical substances cited in National Library of Medicine (NLM) databases, including the TOXNET system. | |
Record name | N-Dodecylacrylamide | |
Source | EPA DSSTox | |
URL | https://comptox.epa.gov/dashboard/DTXSID50164580 | |
Description | DSSTox provides a high quality public chemistry resource for supporting improved predictive toxicology. | |
Record name | N-dodecylacrylamide | |
Source | European Chemicals Agency (ECHA) | |
URL | https://echa.europa.eu/substance-information/-/substanceinfo/100.014.669 | |
Description | The European Chemicals Agency (ECHA) is an agency of the European Union which is the driving force among regulatory authorities in implementing the EU's groundbreaking chemicals legislation for the benefit of human health and the environment as well as for innovation and competitiveness. | |
Explanation | Use of the information, documents and data from the ECHA website is subject to the terms and conditions of this Legal Notice, and subject to other binding limitations provided for under applicable law, the information, documents and data made available on the ECHA website may be reproduced, distributed and/or used, totally or in part, for non-commercial purposes provided that ECHA is acknowledged as the source: "Source: European Chemicals Agency, http://echa.europa.eu/". Such acknowledgement must be included in each copy of the material. ECHA permits and encourages organisations and individuals to create links to the ECHA website under the following cumulative conditions: Links can only be made to webpages that provide a link to the Legal Notice page. | |
Synthesis routes and methods
Procedure details
Retrosynthesis Analysis
AI-Powered Synthesis Planning: Our tool employs the Template_relevance Pistachio, Template_relevance Bkms_metabolic, Template_relevance Pistachio_ringbreaker, Template_relevance Reaxys, Template_relevance Reaxys_biocatalysis model, leveraging a vast database of chemical reactions to predict feasible synthetic routes.
One-Step Synthesis Focus: Specifically designed for one-step synthesis, it provides concise and direct routes for your target compounds, streamlining the synthesis process.
Accurate Predictions: Utilizing the extensive PISTACHIO, BKMS_METABOLIC, PISTACHIO_RINGBREAKER, REAXYS, REAXYS_BIOCATALYSIS database, our tool offers high-accuracy predictions, reflecting the latest in chemical research and data.
Strategy Settings
Precursor scoring | Relevance Heuristic |
---|---|
Min. plausibility | 0.01 |
Model | Template_relevance |
Template Set | Pistachio/Bkms_metabolic/Pistachio_ringbreaker/Reaxys/Reaxys_biocatalysis |
Top-N result to add to graph | 6 |
Feasible Synthetic Routes
Q1: What is the molecular formula and weight of N-Dodecylacrylamide?
A1: The molecular formula of N-Dodecylacrylamide is C15H29NO, and its molecular weight is 251.40 g/mol.
Q2: What spectroscopic data is available for N-Dodecylacrylamide?
A2: Infrared (FTIR) spectroscopy is commonly employed to characterize DDA-containing polymers. FTIR analysis can identify characteristic peaks associated with amide groups (N-H and C=O stretching vibrations) and alkyl chains (C-H stretching and bending vibrations). []
Q3: How does the alkyl chain length in poly(N-alkylacrylamide)s affect their surface activity?
A3: Increasing the alkyl chain length, like in DDA, enhances surface activity in poly(N-alkylacrylamide)s. This translates to a greater ability to lower surface tension and adsorb onto surfaces like polystyrene latex particles. []
Q4: How stable are DDA-based Langmuir-Blodgett (LB) films?
A4: DDA forms robust LB films due to strong hydrogen bonding between amide groups in the polymer backbone. These films demonstrate high stability, even withstanding multiple depositions and remaining intact upon peeling from substrates. [, ]
Q5: How does the presence of N-Dodecylacrylamide in copolymers affect their rheological properties?
A5: Incorporating DDA into copolymers, such as polyacrylamide, significantly enhances their rheological properties, including viscosity and viscoelasticity, particularly under harsh conditions like high salinity and temperature. This is attributed to the hydrophobic associations formed by the dodecyl side chains. [, , , ]
Q6: What are the potential applications of N-Dodecylacrylamide in Enhanced Oil Recovery (EOR)?
A6: DDA-containing associating polymers are investigated for EOR due to their enhanced viscosity and resistance to harsh reservoir conditions. They can improve the mobility ratio during polymer flooding, leading to more efficient oil displacement. [, ]
Q7: Can N-Dodecylacrylamide be used to create functional surfaces?
A7: Yes, DDA LB films can be functionalized with various moieties for specific applications. For example, incorporating reactive groups like terminal amino groups allows for further chemical modification and the creation of pH sensors. []
Q8: How is N-Dodecylacrylamide used in drug delivery research?
A8: Amphiphilic copolymers containing DDA can form nanoparticles that encapsulate hydrophobic drugs. Research shows these nanoparticles have potential as drug carriers, demonstrating the ability to encapsulate and release drugs like griseofulvin. []
Q9: What is the role of N-Dodecylacrylamide in creating electrochemical transistors?
A9: DDA, when combined with conductive polymers like poly(3-hexylthiophene) in LB films, acts as a matrix for organizing the conductive polymer. This results in well-defined layered structures suitable for fabricating electrochemical transistors with promising performance characteristics. [, ]
Q10: How does N-Dodecylacrylamide contribute to the development of oxygen sensors?
A10: DDA, when copolymerized with oxygen-sensitive molecules like platinum porphyrins, enables the creation of porous, superhydrophobic films. These films allow oxygen to permeate from the surrounding environment, facilitating its detection via changes in the luminescence of the incorporated sensor molecules. []
Q11: What types of nanostructures can be formed using N-Dodecylacrylamide-based block copolymers?
A11: DDA-based block copolymers, like those with poly(ethylene glycol), can self-assemble into various nanostructures, including nanodots, nanocylinders, and lamellar structures, depending on factors like annealing conditions and block copolymer composition. [, , ]
Q12: How does humid annealing affect the structure of poly(N-Dodecylacrylamide) films?
A12: Humid annealing induces highly ordered lamellar structures in poly(N-Dodecylacrylamide) films. This ordering arises from the segregation of alkyl side chains and the water-adsorbed main chain, driven by the hydrophobic effect. [, ]
Avertissement et informations sur les produits de recherche in vitro
Veuillez noter que tous les articles et informations sur les produits présentés sur BenchChem sont destinés uniquement à des fins informatives. Les produits disponibles à l'achat sur BenchChem sont spécifiquement conçus pour des études in vitro, qui sont réalisées en dehors des organismes vivants. Les études in vitro, dérivées du terme latin "in verre", impliquent des expériences réalisées dans des environnements de laboratoire contrôlés à l'aide de cellules ou de tissus. Il est important de noter que ces produits ne sont pas classés comme médicaments et n'ont pas reçu l'approbation de la FDA pour la prévention, le traitement ou la guérison de toute condition médicale, affection ou maladie. Nous devons souligner que toute forme d'introduction corporelle de ces produits chez les humains ou les animaux est strictement interdite par la loi. Il est essentiel de respecter ces directives pour assurer la conformité aux normes légales et éthiques en matière de recherche et d'expérimentation.