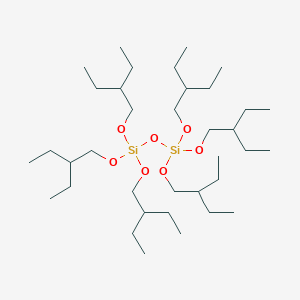
Hexakis(2-ethylbutoxy)disiloxane
- Cliquez sur DEMANDE RAPIDE pour recevoir un devis de notre équipe d'experts.
- Avec des produits de qualité à un prix COMPÉTITIF, vous pouvez vous concentrer davantage sur votre recherche.
Vue d'ensemble
Description
Hexakis(2-ethylbutoxy)disiloxane is a heterocyclic organic compound with the molecular formula C36H78O7Si2 and a molecular weight of 679.17 . It is also known by other names such as HEXA (2-ETHYLBUTOXY)DISILOXANE and tris (2-ethylbutoxy)- [tris (2-ethylbutoxy)silyloxy]silane .
Molecular Structure Analysis
The molecular structure of Hexakis(2-ethylbutoxy)disiloxane consists of 36 carbon atoms, 78 hydrogen atoms, 7 oxygen atoms, and 2 silicon atoms . The exact structure is not provided in the search results.Safety And Hazards
The safety data sheet for Hexakis(2-ethylbutoxy)disiloxane suggests that in case of inhalation, the victim should be moved into fresh air and given oxygen if breathing is difficult . If the chemical comes into contact with the skin, contaminated clothing should be removed immediately and the skin should be washed off with soap and plenty of water . In case of eye contact, the eyes should be rinsed with pure water for at least 15 minutes . If ingested, the mouth should be rinsed with water and vomiting should not be induced . In all cases, a doctor should be consulted immediately .
Propriétés
IUPAC Name |
tris(2-ethylbutyl) tris(2-ethylbutoxy)silyl silicate |
Source
|
---|---|---|
Source | PubChem | |
URL | https://pubchem.ncbi.nlm.nih.gov | |
Description | Data deposited in or computed by PubChem | |
InChI |
InChI=1S/C36H78O7Si2/c1-13-31(14-2)25-37-44(38-26-32(15-3)16-4,39-27-33(17-5)18-6)43-45(40-28-34(19-7)20-8,41-29-35(21-9)22-10)42-30-36(23-11)24-12/h31-36H,13-30H2,1-12H3 |
Source
|
Source | PubChem | |
URL | https://pubchem.ncbi.nlm.nih.gov | |
Description | Data deposited in or computed by PubChem | |
InChI Key |
LZABKCXEHHOOHI-UHFFFAOYSA-N |
Source
|
Source | PubChem | |
URL | https://pubchem.ncbi.nlm.nih.gov | |
Description | Data deposited in or computed by PubChem | |
Canonical SMILES |
CCC(CC)CO[Si](OCC(CC)CC)(OCC(CC)CC)O[Si](OCC(CC)CC)(OCC(CC)CC)OCC(CC)CC |
Source
|
Source | PubChem | |
URL | https://pubchem.ncbi.nlm.nih.gov | |
Description | Data deposited in or computed by PubChem | |
Molecular Formula |
C36H78O7Si2 |
Source
|
Source | PubChem | |
URL | https://pubchem.ncbi.nlm.nih.gov | |
Description | Data deposited in or computed by PubChem | |
DSSTOX Substance ID |
DTXSID20300892 |
Source
|
Record name | HEXA(2-ETHYLBUTOXY)DISILOXANE | |
Source | EPA DSSTox | |
URL | https://comptox.epa.gov/dashboard/DTXSID20300892 | |
Description | DSSTox provides a high quality public chemistry resource for supporting improved predictive toxicology. | |
Molecular Weight |
679.2 g/mol |
Source
|
Source | PubChem | |
URL | https://pubchem.ncbi.nlm.nih.gov | |
Description | Data deposited in or computed by PubChem | |
Product Name |
Hexakis(2-ethylbutoxy)disiloxane | |
CAS RN |
1476-03-5 |
Source
|
Record name | NSC139840 | |
Source | DTP/NCI | |
URL | https://dtp.cancer.gov/dtpstandard/servlet/dwindex?searchtype=NSC&outputformat=html&searchlist=139840 | |
Description | The NCI Development Therapeutics Program (DTP) provides services and resources to the academic and private-sector research communities worldwide to facilitate the discovery and development of new cancer therapeutic agents. | |
Explanation | Unless otherwise indicated, all text within NCI products is free of copyright and may be reused without our permission. Credit the National Cancer Institute as the source. | |
Record name | HEXA(2-ETHYLBUTOXY)DISILOXANE | |
Source | EPA DSSTox | |
URL | https://comptox.epa.gov/dashboard/DTXSID20300892 | |
Description | DSSTox provides a high quality public chemistry resource for supporting improved predictive toxicology. | |
Avertissement et informations sur les produits de recherche in vitro
Veuillez noter que tous les articles et informations sur les produits présentés sur BenchChem sont destinés uniquement à des fins informatives. Les produits disponibles à l'achat sur BenchChem sont spécifiquement conçus pour des études in vitro, qui sont réalisées en dehors des organismes vivants. Les études in vitro, dérivées du terme latin "in verre", impliquent des expériences réalisées dans des environnements de laboratoire contrôlés à l'aide de cellules ou de tissus. Il est important de noter que ces produits ne sont pas classés comme médicaments et n'ont pas reçu l'approbation de la FDA pour la prévention, le traitement ou la guérison de toute condition médicale, affection ou maladie. Nous devons souligner que toute forme d'introduction corporelle de ces produits chez les humains ou les animaux est strictement interdite par la loi. Il est essentiel de respecter ces directives pour assurer la conformité aux normes légales et éthiques en matière de recherche et d'expérimentation.