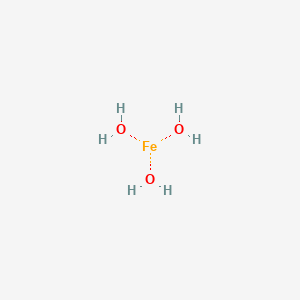
Hydroxyde ferrique
Vue d'ensemble
Description
Ferric hydroxide, also known as iron(III) hydroxide, is a chemical compound with the formula Fe(OH)₃. It is commonly recognized as a yellowish-brown or reddish-brown precipitate. This compound is practically insoluble in water but dissolves readily in acids to produce iron(III) salts. Ferric hydroxide naturally occurs in the environment as the mineral goethite and is a primary component in the formation of rust when iron reacts with water and oxygen .
Applications De Recherche Scientifique
Arsenic Removal
Ferric hydroxide is widely recognized for its effectiveness in removing arsenic from drinking water. Granular ferric hydroxide (GFH) has been developed specifically for this purpose. It operates efficiently in fixed bed reactors, exhibiting a high adsorption capacity that can treat 30,000 to 40,000 bed volumes before exceeding the WHO standard of 10 μg/L for arsenic . The process generates solid waste enriched with arsenic, which poses disposal challenges but remains a viable option for small water facilities.
Heavy Metal Removal
In addition to arsenic, ferric hydroxide is effective in removing other heavy metals such as chromium and lead from contaminated water sources. Its application spans various settings, including wastewater treatment plants and industrial effluents . The compound's ability to precipitate heavy metals makes it a crucial component in environmental cleanup strategies.
Heavy Metal | Removal Efficiency | Application |
---|---|---|
Arsenic | Up to 99% | Drinking water treatment |
Chromium | High | Industrial wastewater treatment |
Lead | High | Contaminated groundwater remediation |
Food Fortification
Ferric hydroxide has been explored as a source of iron in food fortification programs. Studies indicate its potential to enhance iron bioavailability when used in conjunction with other compounds like EDTA . This application is particularly significant in addressing micronutrient deficiencies in populations at risk of anemia.
Case Study: Iron Fortification
A notable case study involved the use of ferric hydroxide in the Philippines, where it was integrated into food products to combat iron deficiency anemia. The initiative demonstrated improved hemoglobin levels among participants consuming fortified foods .
Soil Amendment
Residues from water treatment processes using ferric hydroxide have been utilized as soil amendments. However, recent findings suggest that these residues can lock phosphorus in the soil, reducing its availability for plant nutrition by over 70% . This highlights the need for careful management of ferric sludge in agricultural practices.
Soil Parameter | Before Application | After Application |
---|---|---|
Mobile Phosphorus (Pm) | High | Significantly Reduced |
Extractable Phosphorus (Pe) | High | Significantly Reduced |
Environmental Impact
The environmental implications of using ferric hydroxide residues are significant. While they can improve soil structure and reduce contaminants, their effect on nutrient cycling must be further studied to ensure sustainable agricultural practices .
Industrial Applications
Ferric hydroxide is also employed in various industrial processes:
Mécanisme D'action
Target of Action
Ferric hydroxide is primarily targeted towards the treatment and prevention of iron deficiency anemia . Iron deficiency anemia is a condition where the body lacks enough iron to produce hemoglobin, the protein in red blood cells that carries oxygen to the body’s tissues. Ferric hydroxide, as an iron supplement, helps replenish the body’s iron stores and aids in the production of hemoglobin .
Mode of Action
The iron ions are then transported as a complex with transferrin to target cells including erythroid precursor cells . The iron is then incorporated into hemoglobin as the cells mature into red blood cells .
Biochemical Pathways
Ferric hydroxide plays a crucial role in the iron metabolic pathway. It contributes to the replenishment of iron stores in the body, which is essential for the synthesis of hemoglobin. Hemoglobin, in turn, is vital for the transport of oxygen in the body. Therefore, ferric hydroxide indirectly influences the oxygen transport pathway and the overall oxygenation of the body’s tissues .
Pharmacokinetics
This is why it is often administered intravenously in the form of ferric carboxymaltose, a colloidal iron (III) hydroxide in complex with carboxymaltose, a carbohydrate polymer that releases iron .
Result of Action
The primary result of ferric hydroxide administration is the correction of iron deficiency and the prevention or treatment of iron deficiency anemia . By replenishing the body’s iron stores, ferric hydroxide aids in the production of hemoglobin, thereby improving the oxygen-carrying capacity of the blood. This can lead to an improvement in symptoms associated with iron deficiency anemia, such as fatigue and weakness .
Action Environment
The action of ferric hydroxide can be influenced by various environmental factors. For instance, the presence of other substances in the gastrointestinal tract can affect the absorption of ferric hydroxide. Certain substances, such as tannins found in tea and coffee, can inhibit iron absorption, while others, like vitamin C, can enhance it . Furthermore, the pH of the environment can also affect the solubility and hence the bioavailability of ferric hydroxide . In alkaline conditions, ferric hydroxide tends to precipitate, reducing its bioavailability .
Analyse Biochimique
Biochemical Properties
Ferric hydroxide interacts with various enzymes, proteins, and other biomolecules. For instance, it is involved in the oxidation of iron by bacterial metabolic systems . The ferric ions produced by biological actions also act as a catalyst for the oxidation of toxic metalloids .
Cellular Effects
Ferric hydroxide influences cell function by affecting the cycling of iron and the concentrations of hazardous metals . It also has an impact on cell signaling pathways, gene expression, and cellular metabolism .
Molecular Mechanism
At the molecular level, ferric hydroxide exerts its effects through binding interactions with biomolecules and changes in gene expression . It also plays a role in enzyme inhibition or activation .
Temporal Effects in Laboratory Settings
In laboratory settings, the effects of ferric hydroxide change over time. Information on the product’s stability, degradation, and long-term effects on cellular function can be observed in in vitro or in vivo studies .
Dosage Effects in Animal Models
The effects of ferric hydroxide vary with different dosages in animal models . Studies have observed threshold effects, as well as toxic or adverse effects at high doses .
Metabolic Pathways
Ferric hydroxide is involved in various metabolic pathways. It interacts with enzymes or cofactors and affects metabolic flux or metabolite levels .
Transport and Distribution
Ferric hydroxide is transported and distributed within cells and tissues . It interacts with transporters or binding proteins and affects its localization or accumulation .
Subcellular Localization
The subcellular localization of ferric hydroxide and its effects on activity or function are significant . It could include any targeting signals or post-translational modifications that direct it to specific compartments or organelles .
Méthodes De Préparation
Synthetic Routes and Reaction Conditions: Ferric hydroxide is most commonly synthesized in the laboratory by adding a solution of an iron(III) salt, such as iron(III) chloride or iron(III) nitrate, to a strong base like sodium hydroxide. The reaction is as follows: [ \text{Fe}^{3+} + 3\text{OH}^- \rightarrow \text{Fe(OH)}_3 ] This process results in the formation of a precipitate of ferric hydroxide .
Industrial Production Methods: In industrial settings, ferric hydroxide can be produced by the hydrolysis of ferric chloride with boiling water. The reaction is: [ \text{FeCl}_3 + 3\text{H}_2\text{O} \rightarrow \text{Fe(OH)}_3 + 3\text{HCl} ] The hydrochloric acid formed during the hydrolysis must be removed through dialysis to stabilize the ferric hydroxide sol .
Analyse Des Réactions Chimiques
Types of Reactions: Ferric hydroxide undergoes various chemical reactions, including:
Oxidation: Ferric hydroxide can be oxidized to form iron(III) oxide (Fe₂O₃).
Reduction: It can be reduced to iron(II) hydroxide (Fe(OH)₂) under certain conditions.
Substitution: Ferric hydroxide can react with acids to form iron(III) salts and water.
Common Reagents and Conditions:
Oxidation: Exposure to air or other oxidizing agents.
Reduction: Reaction with reducing agents such as hydrogen gas.
Substitution: Reaction with acids like hydrochloric acid.
Major Products:
Oxidation: Iron(III) oxide (Fe₂O₃).
Reduction: Iron(II) hydroxide (Fe(OH)₂).
Substitution: Iron(III) salts (e.g., iron(III) chloride) and water.
Comparaison Avec Des Composés Similaires
Ferric hydroxide can be compared with other iron compounds such as:
Iron(III) oxide (Fe₂O₃):
Iron(III) oxide-hydroxide (FeO(OH)):
Iron(II) hydroxide (Fe(OH)₂): A reduced form of ferric hydroxide, it is less stable and more reactive
Ferric hydroxide is unique in its ability to act as a precursor to various iron compounds and its extensive use in medical and industrial applications.
Activité Biologique
Ferric hydroxide (Fe(OH)₃) is a compound that plays a significant role in various biological and environmental processes. It is primarily known for its applications in water treatment, iron supplementation, and as a sorbent for contaminants. This article explores the biological activity of ferric hydroxide, supported by case studies, research findings, and data tables.
Ferric hydroxide is an insoluble compound that forms under alkaline conditions when ferrous iron (Fe²⁺) is oxidized to ferric iron (Fe³⁺). The precipitation of ferric hydroxide can occur through biological processes, particularly by iron-oxidizing bacteria like Thiobacillus ferrooxidans, which utilize Fe²⁺ as an energy source. This biological oxidation results in the formation of ferric hydroxides that can scavenge other metal cations from the environment .
Iron Supplementation
Ferric hydroxide is commonly used as an iron supplement, particularly in patients with iron deficiency anemia. Research indicates that nano-formulations of ferric hydroxide exhibit improved bioavailability compared to traditional forms. A study demonstrated that unmodified ferric hydroxide had a relative bioavailability (RBV) five times lower than ligand-modified nano-Fe(III) materials in humans . This highlights the importance of formulation in enhancing the absorption and utilization of iron.
Adsorption of Contaminants
Ferric hydroxide has significant adsorption properties, making it effective in removing contaminants from water. For instance, it has been used to mitigate arsenic concentrations in drinking water, particularly in mining areas where arsenic contamination is prevalent. A case study from Kitwe, Zambia, showed that using ferric hydroxide soil samples effectively reduced arsenic levels, contributing to lower incidences of cancer-related diseases among residents .
Case Studies
- Water Treatment : A study on European drinking water treatment plants highlighted the effectiveness of ferric hydroxide in phosphorus immobilization. Engineers expressed confidence in its ability to manage ferrous sludge from drinking water clarification processes .
- Dye Removal : A comparative study investigated the efficiency of ferric hydroxide in removing synthetic dyes from wastewater. The study found that ferric hydroxide achieved an average removal rate of 43.11%, demonstrating its utility in environmental cleanup efforts .
Table 1: Summary of Ferric Hydroxide Applications
Application | Effectiveness | Reference |
---|---|---|
Iron Supplementation | Enhanced bioavailability | |
Arsenic Mitigation | Significant reduction in levels | |
Dye Removal | 43.11% average removal efficiency |
Cellular Uptake Studies
Research on cellular uptake has shown that ferric hydroxide's solubility affects its absorption in biological systems. In vitro studies indicated that the cellular utilization of iron closely correlates with the amount absorbed by cells, emphasizing the need for optimal formulation to enhance bioavailability .
Propriétés
IUPAC Name |
iron;trihydrate | |
---|---|---|
Source | PubChem | |
URL | https://pubchem.ncbi.nlm.nih.gov | |
Description | Data deposited in or computed by PubChem | |
InChI |
InChI=1S/Fe.3H2O/h;3*1H2 | |
Source | PubChem | |
URL | https://pubchem.ncbi.nlm.nih.gov | |
Description | Data deposited in or computed by PubChem | |
InChI Key |
FLTRNWIFKITPIO-UHFFFAOYSA-N | |
Source | PubChem | |
URL | https://pubchem.ncbi.nlm.nih.gov | |
Description | Data deposited in or computed by PubChem | |
Canonical SMILES |
O.O.O.[Fe] | |
Source | PubChem | |
URL | https://pubchem.ncbi.nlm.nih.gov | |
Description | Data deposited in or computed by PubChem | |
Molecular Formula |
FeH6O3 | |
Source | PubChem | |
URL | https://pubchem.ncbi.nlm.nih.gov | |
Description | Data deposited in or computed by PubChem | |
DSSTOX Substance ID |
DTXSID5051652 | |
Record name | Ferric hydroxide | |
Source | EPA DSSTox | |
URL | https://comptox.epa.gov/dashboard/DTXSID5051652 | |
Description | DSSTox provides a high quality public chemistry resource for supporting improved predictive toxicology. | |
Molecular Weight |
109.89 g/mol | |
Source | PubChem | |
URL | https://pubchem.ncbi.nlm.nih.gov | |
Description | Data deposited in or computed by PubChem | |
Physical Description |
Liquid; Water or Solvent Wet Solid, Red to brown solid; Practically insoluble in water; [Merck Index] Red-brown crystals; [Sigma-Aldrich MSDS] | |
Record name | Iron hydroxide (Fe(OH)3) | |
Source | EPA Chemicals under the TSCA | |
URL | https://www.epa.gov/chemicals-under-tsca | |
Description | EPA Chemicals under the Toxic Substances Control Act (TSCA) collection contains information on chemicals and their regulations under TSCA, including non-confidential content from the TSCA Chemical Substance Inventory and Chemical Data Reporting. | |
Record name | Ferric hydroxide | |
Source | Haz-Map, Information on Hazardous Chemicals and Occupational Diseases | |
URL | https://haz-map.com/Agents/14952 | |
Description | Haz-Map® is an occupational health database designed for health and safety professionals and for consumers seeking information about the adverse effects of workplace exposures to chemical and biological agents. | |
Explanation | Copyright (c) 2022 Haz-Map(R). All rights reserved. Unless otherwise indicated, all materials from Haz-Map are copyrighted by Haz-Map(R). No part of these materials, either text or image may be used for any purpose other than for personal use. Therefore, reproduction, modification, storage in a retrieval system or retransmission, in any form or by any means, electronic, mechanical or otherwise, for reasons other than personal use, is strictly prohibited without prior written permission. | |
CAS No. |
1309-33-7 | |
Record name | Ferric hydroxide | |
Source | ChemIDplus | |
URL | https://pubchem.ncbi.nlm.nih.gov/substance/?source=chemidplus&sourceid=0001309337 | |
Description | ChemIDplus is a free, web search system that provides access to the structure and nomenclature authority files used for the identification of chemical substances cited in National Library of Medicine (NLM) databases, including the TOXNET system. | |
Record name | Iron hydroxide (Fe(OH)3) | |
Source | EPA Chemicals under the TSCA | |
URL | https://www.epa.gov/chemicals-under-tsca | |
Description | EPA Chemicals under the Toxic Substances Control Act (TSCA) collection contains information on chemicals and their regulations under TSCA, including non-confidential content from the TSCA Chemical Substance Inventory and Chemical Data Reporting. | |
Record name | Ferric hydroxide | |
Source | EPA DSSTox | |
URL | https://comptox.epa.gov/dashboard/DTXSID5051652 | |
Description | DSSTox provides a high quality public chemistry resource for supporting improved predictive toxicology. | |
Record name | Diiron trioxide hydrate | |
Source | European Chemicals Agency (ECHA) | |
URL | https://echa.europa.eu/substance-information/-/substanceinfo/100.013.788 | |
Description | The European Chemicals Agency (ECHA) is an agency of the European Union which is the driving force among regulatory authorities in implementing the EU's groundbreaking chemicals legislation for the benefit of human health and the environment as well as for innovation and competitiveness. | |
Explanation | Use of the information, documents and data from the ECHA website is subject to the terms and conditions of this Legal Notice, and subject to other binding limitations provided for under applicable law, the information, documents and data made available on the ECHA website may be reproduced, distributed and/or used, totally or in part, for non-commercial purposes provided that ECHA is acknowledged as the source: "Source: European Chemicals Agency, http://echa.europa.eu/". Such acknowledgement must be included in each copy of the material. ECHA permits and encourages organisations and individuals to create links to the ECHA website under the following cumulative conditions: Links can only be made to webpages that provide a link to the Legal Notice page. | |
Retrosynthesis Analysis
AI-Powered Synthesis Planning: Our tool employs the Template_relevance Pistachio, Template_relevance Bkms_metabolic, Template_relevance Pistachio_ringbreaker, Template_relevance Reaxys, Template_relevance Reaxys_biocatalysis model, leveraging a vast database of chemical reactions to predict feasible synthetic routes.
One-Step Synthesis Focus: Specifically designed for one-step synthesis, it provides concise and direct routes for your target compounds, streamlining the synthesis process.
Accurate Predictions: Utilizing the extensive PISTACHIO, BKMS_METABOLIC, PISTACHIO_RINGBREAKER, REAXYS, REAXYS_BIOCATALYSIS database, our tool offers high-accuracy predictions, reflecting the latest in chemical research and data.
Strategy Settings
Precursor scoring | Relevance Heuristic |
---|---|
Min. plausibility | 0.01 |
Model | Template_relevance |
Template Set | Pistachio/Bkms_metabolic/Pistachio_ringbreaker/Reaxys/Reaxys_biocatalysis |
Top-N result to add to graph | 6 |
Feasible Synthetic Routes
Avertissement et informations sur les produits de recherche in vitro
Veuillez noter que tous les articles et informations sur les produits présentés sur BenchChem sont destinés uniquement à des fins informatives. Les produits disponibles à l'achat sur BenchChem sont spécifiquement conçus pour des études in vitro, qui sont réalisées en dehors des organismes vivants. Les études in vitro, dérivées du terme latin "in verre", impliquent des expériences réalisées dans des environnements de laboratoire contrôlés à l'aide de cellules ou de tissus. Il est important de noter que ces produits ne sont pas classés comme médicaments et n'ont pas reçu l'approbation de la FDA pour la prévention, le traitement ou la guérison de toute condition médicale, affection ou maladie. Nous devons souligner que toute forme d'introduction corporelle de ces produits chez les humains ou les animaux est strictement interdite par la loi. Il est essentiel de respecter ces directives pour assurer la conformité aux normes légales et éthiques en matière de recherche et d'expérimentation.