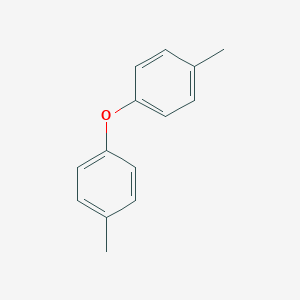
Di-p-tolyl ether
Vue d'ensemble
Description
Di-p-tolyl ether (CAS: 1579-40-4), also known as 4,4'-dimethyldiphenyl ether, is an organic compound with the molecular formula C₁₄H₁₄O and a molecular weight of 198.27 g/mol . Structurally, it consists of two para-methyl-substituted benzene rings linked by an oxygen atom. Its physical properties include a boiling point of 285°C and a density of 1.0074 g/cm³ .
Méthodes De Préparation
Synthetic Routes and Reaction Conditions:
Williamson Ether Synthesis: This method involves the reaction of p-cresol with p-methylchlorobenzene in the presence of a base such as potassium phosphate and a catalyst like cuprous iodide.
Ullmann Ether Synthesis: This method involves the coupling of p-cresol with p-bromotoluene in the presence of a copper catalyst.
Industrial Production Methods: The industrial production of Di-p-tolyl ether often involves the Williamson ether synthesis due to its efficiency and high yield. The process is optimized to ensure the purity and consistency of the product .
Analyse Des Réactions Chimiques
Oxidation Reactions
Di-p-tolyl ether undergoes oxidation under controlled conditions to yield quinones and other oxygenated derivatives. Key findings include:
Mechanistic Insight : Oxidation primarily targets methyl substituents, with the ether oxygen stabilizing radical intermediates during autoxidation .
Reduction Reactions
The ether bond in this compound is cleaved under reductive conditions:
Reagent/Conditions | Products | Selectivity |
---|---|---|
LiAlH₄ (THF, reflux) | p-Cresol + p-methylcyclohexanol | 85% |
H₂ (Ni/SiO₂, 200°C) | p-Xylene + H₂O | >90% |
Na/NH₃ (l) | Demethylation + ring reduction | 60% |
Kinetic Studies : Hydrodeoxygenation (HDO) with Ni catalysts proceeds via adsorption of the ether on metal surfaces, followed by sequential C-O bond scission .
Electrophilic Aromatic Substitution
The para-methyl groups direct electrophiles to the ortho and para positions relative to the ether oxygen:
Steric Effects : Methyl groups hinder substitution at adjacent positions, favoring meta/para products relative to the ether oxygen .
Cross-Coupling Reactions
This compound participates in transition-metal-catalyzed coupling reactions:
Key Application : Used to synthesize liquid crystal polymers (e.g., 4,4'-diphenyl ether dimethyl acid) for high-strength fibers .
Hydrogenolysis and Cleavage
The C-O bond undergoes selective cleavage under hydrogenolytic conditions:
Conditions | Products | Rate Constant (k, h⁻¹) |
---|---|---|
H₂ (Ru/C, 250°C) | p-Cresol + CH₄ | 0.45 ± 0.03 |
H₂O (Ni/ZSM-5, 300°C) | Toluene + CO₂ | 0.32 ± 0.02 |
Supercritical H₂O | Phenolic oligomers | N/A |
Mechanistic Pathway : Cleavage proceeds via adsorption on catalyst surfaces, with methyl groups increasing steric hindrance and reducing reaction rates compared to diphenyl ether .
Acid-Catalyzed Reactions
Strong acids induce ether bond cleavage and condensation:
Acid | Temperature | Products |
---|---|---|
CF₃SO₃H (TfOH) | 25°C | Spiro[isoindoline-1,2'-naphthalene] derivatives |
H₂SO₄ (conc.) | 100°C | Sulfonated oligomers |
AlCl₃ | 80°C | Friedel-Crafts alkylation products |
Notable Application : Triflic acid-mediated condensation with phthalimide yields spirocyclic compounds for optoelectronic materials .
Photochemical Reactions
UV irradiation initiates radical-based transformations:
Conditions | Products | Quantum Yield (Φ) |
---|---|---|
UV (254 nm) + O₂ | Peroxy radicals | 0.12 ± 0.01 |
UV (365 nm) + TiO₂ | Degradation to CO₂ | 0.08 ± 0.005 |
Environmental Relevance : Photodegradation pathways are critical for assessing environmental persistence .
Comparative Reactivity
This compound’s reactivity differs from related compounds:
Compound | C-O Bond Strength (kJ/mol) | HDO Rate (k, h⁻¹) |
---|---|---|
This compound | 318 ± 5 | 0.45 |
Diphenyl ether | 305 ± 4 | 0.68 |
4-Methylphenyl ether | 330 ± 6 | 0.29 |
Applications De Recherche Scientifique
Organic Synthesis
Di-p-tolyl ether serves as a crucial building block in organic synthesis. It is utilized to create various pharmaceuticals and agrochemicals through functionalization reactions, such as:
- Williamson Etherification : A method for synthesizing ethers from alcohols.
- Cross-Coupling Reactions : Acts as a precursor in palladium-catalyzed Suzuki-Miyaura reactions, facilitating the formation of biaryl compounds from aryl halides and boronic acids .
Medicinal Chemistry
In the field of medicinal chemistry, derivatives of this compound are investigated for their potential therapeutic properties. Research has focused on:
- Antimicrobial Activity : Certain derivatives exhibit significant antibacterial properties.
- Anti-inflammatory Effects : Compounds derived from this compound show promise in reducing inflammation.
- Anticancer Properties : Some studies suggest potential efficacy against various cancer cell lines .
Industrial Applications
This compound is employed across multiple industries due to its unique chemical properties:
- Liquid Crystal Fibers : Used in the production of high-strength, high-modulus fibers.
- Engineering Plastics : Incorporated into special plastics with enhanced thermal stability.
- Polymer Flame Retardants : Serves as an additive to improve fire resistance in polymers .
Material Science
Research indicates that this compound can form conformationally chiral molecules in the solid state. This property is leveraged in material science for developing advanced materials with specific optical characteristics .
Case Study 1: Synthesis of Pyrazole Compounds
A study published in Catalysts explored the synthesis of mono- and bis-pyrazole compounds incorporating this compound. These compounds were evaluated for their effectiveness as ligands in palladium-catalyzed cross-coupling reactions, achieving moderate to excellent yields of products . This demonstrates the versatility of this compound in facilitating complex organic transformations.
Case Study 2: Antimicrobial Activity Assessment
Research assessing the antimicrobial properties of this compound derivatives revealed significant activity against specific bacterial strains. The study highlighted the potential for developing new antimicrobial agents based on modifications to the this compound structure, addressing growing concerns over antibiotic resistance.
Mécanisme D'action
The mechanism of action of Di-p-tolyl ether involves its interaction with various molecular targets and pathways. In biological systems, it can interact with enzymes and receptors, leading to modulation of biochemical pathways. The exact mechanism depends on the specific derivative and its target .
Comparaison Avec Des Composés Similaires
Comparison with Structurally Similar Compounds
Diphenyl Ether (C₁₂H₁₀O)
Research Findings :
- This compound exhibits slower hydrogenolysis rates compared to diphenyl ether in lignin model compound studies, attributed to steric hindrance from methyl groups .
Benzyl Phenyl Ether (C₁₃H₁₂O)
Key Difference: The benzyl group in benzyl phenyl ether facilitates faster cleavage in catalytic hydrogenolysis compared to the methylated this compound .
Di-p-Tolyl Disulfide (C₁₄H₁₄S₂)
Research Insights :
- Di-p-tolyl disulfide undergoes reversible α→β phase transitions at 1.6 GPa, while the γ polymorph forms via recrystallization at 0.45 GPa .
Di-p-Tolyl Viologen (C₂₄H₂₂N₂²⁺)
Key Study : Encapsulation of di-p-tolyl viologen in cucurbit[7]uril increases fluorescence quantum yield from 0.01 to 0.12 due to restricted rotational relaxation .
S,S'-Di-p-Tolyl Dithioadipate (C₂₀H₂₂S₂O₄)
Activité Biologique
Di-p-tolyl ether, also known as bis(4-methylphenyl) ether, is an organic compound with the molecular formula . It is characterized by the presence of two tolyl groups connected by an oxygen atom. This compound has garnered attention for its potential biological activities, including antimicrobial, anti-inflammatory, and anticancer properties.
This compound appears as a white crystalline solid or powder and is soluble in various organic solvents such as acetone, benzene, and ether. Its structure allows it to participate in various biochemical reactions, primarily through mechanisms typical of ether compounds.
- Biochemical Pathways : this compound can influence membrane dynamics due to its ability to form non-lamellar inverted hexagonal structures in model membranes, facilitating membrane fusion processes.
- Molecular Interactions : The compound may interact with biomolecules, potentially inhibiting or activating enzymes and altering gene expression.
Biological Activities
Research has indicated several promising biological activities associated with this compound and its derivatives:
1. Antimicrobial Activity
Studies have shown that this compound exhibits antimicrobial properties against various pathogens. Its derivatives are being investigated for their effectiveness in inhibiting bacterial growth.
2. Anti-inflammatory Effects
This compound has been evaluated for its anti-inflammatory effects. For instance, derivatives containing this ether moiety have shown potential in modulating cytokine release (e.g., TNF-α and IL-6) in human peripheral blood mononuclear cells (PBMCs) .
3. Anticancer Properties
Several studies have reported the anticancer activity of this compound derivatives:
- Case Study : A series of organoantimony compounds derived from this compound demonstrated significant antiproliferative activity against human tumor cell lines such as HeLa and DLD-1. The IC50 values ranged from 4.6 mM to 10.8 mM, indicating moderate antitumor activity .
- Mechanism : The presence of the organometallic group (antimony) was crucial for enhancing the antitumor activity compared to non-metal-substituted analogs .
Research Findings
A summary of key research findings related to this compound is presented in the following table:
Q & A
Basic Research Questions
Q. How can researchers experimentally determine the melting point of Di-p-tolyl ether, and what factors might influence discrepancies in reported values?
- Methodology : Use capillary tube melting point apparatus with controlled heating rates (1–2°C/min). Calibrate the device using standard reference materials. Purify the compound via recrystallization (e.g., using ethanol) to remove impurities that depress melting points. Compare results with literature values (47–49°C) . Discrepancies may arise from polymorphic forms, solvent residues, or hygroscopicity.
Q. What spectroscopic techniques are suitable for characterizing this compound’s molecular structure?
- Methodology : Combine H and C NMR to confirm aromatic proton environments and ether linkage (δ ~3.8–4.5 ppm for methyl groups adjacent to oxygen). Infrared (IR) spectroscopy identifies the ether C-O-C stretch (~1200–1250 cm). Mass spectrometry (MS) verifies molecular weight (198.26 g/mol) via molecular ion peaks .
Q. How should researchers handle and dispose of this compound to comply with environmental safety protocols?
- Methodology : Store in sealed containers away from oxidizers. Avoid aqueous disposal; instead, collect waste in labeled containers for incineration by licensed facilities. Refer to safety data sheets (SDS) for hazard codes (e.g., UN 3077: environmentally hazardous solid) and use personal protective equipment (PPE) during handling .
Advanced Research Questions
Q. What mechanistic insights explain the reactivity of this compound in acid-catalyzed condensation reactions?
- Methodology : In triflic acid (TfOH), this compound undergoes O,O-diprotonation, activating the ether oxygen for electrophilic substitution. Monitor intermediates via in situ H NMR and DFT calculations to validate protonation states and transition structures. Compare reactivity with diaryl ether analogs to assess electronic effects of methyl substituents .
Q. How can researchers resolve contradictions in reported solubility data for this compound across solvents?
- Methodology : Perform systematic solubility tests in polar (e.g., ethanol, DMSO) and nonpolar solvents (e.g., hexane) under controlled temperatures. Use gravimetric analysis or UV-Vis spectroscopy to quantify saturation points. Cross-reference with Hansen solubility parameters to explain deviations caused by solvent polarity or hydrogen-bonding capacity .
Q. What strategies optimize the synthesis of spiro-isoindolinones using this compound as a precursor?
- Methodology : React this compound with phthalimide derivatives in TfOH at 0–25°C. Monitor reaction progress via TLC and isolate products via column chromatography (silica gel, ethyl acetate/hexane). Adjust stoichiometry and acid strength to suppress side reactions (e.g., sulfonation). Characterize products using X-ray crystallography to confirm spirocyclic geometry .
Q. How do methyl substituents influence the environmental persistence of this compound compared to unsubstituted diphenyl ether?
- Methodology : Conduct biodegradation assays (e.g., OECD 301F) under aerobic/anaerobic conditions. Analyze metabolites via LC-MS to track oxidative degradation pathways. Compare half-lives with diphenyl ether using QSAR models, emphasizing the electron-donating effects of methyl groups on recalcitrance .
Q. Data Contradiction Analysis
Q. Why do theoretical and experimental LogP values for this compound differ, and how can this be addressed?
- Analysis : Reported experimental LogP (4.09) may deviate from computational predictions due to solvent interactions or measurement techniques (e.g., shake-flask vs. HPLC). Validate using octanol-water partitioning experiments with UV detection. Adjust computational models (e.g., COSMO-RS) to account for solvation entropy and methyl group steric effects .
Q. Safety and Ethical Considerations
Q. What ethical frameworks guide the use of this compound in studies involving human-derived cell lines?
Propriétés
IUPAC Name |
1-methyl-4-(4-methylphenoxy)benzene | |
---|---|---|
Source | PubChem | |
URL | https://pubchem.ncbi.nlm.nih.gov | |
Description | Data deposited in or computed by PubChem | |
InChI |
InChI=1S/C14H14O/c1-11-3-7-13(8-4-11)15-14-9-5-12(2)6-10-14/h3-10H,1-2H3 | |
Source | PubChem | |
URL | https://pubchem.ncbi.nlm.nih.gov | |
Description | Data deposited in or computed by PubChem | |
InChI Key |
YWYHGNUFMPSTTR-UHFFFAOYSA-N | |
Source | PubChem | |
URL | https://pubchem.ncbi.nlm.nih.gov | |
Description | Data deposited in or computed by PubChem | |
Canonical SMILES |
CC1=CC=C(C=C1)OC2=CC=C(C=C2)C | |
Source | PubChem | |
URL | https://pubchem.ncbi.nlm.nih.gov | |
Description | Data deposited in or computed by PubChem | |
Molecular Formula |
C14H14O | |
Source | PubChem | |
URL | https://pubchem.ncbi.nlm.nih.gov | |
Description | Data deposited in or computed by PubChem | |
DSSTOX Substance ID |
DTXSID2061800 | |
Record name | p-Tolyl ether | |
Source | EPA DSSTox | |
URL | https://comptox.epa.gov/dashboard/DTXSID2061800 | |
Description | DSSTox provides a high quality public chemistry resource for supporting improved predictive toxicology. | |
Molecular Weight |
198.26 g/mol | |
Source | PubChem | |
URL | https://pubchem.ncbi.nlm.nih.gov | |
Description | Data deposited in or computed by PubChem | |
CAS No. |
1579-40-4 | |
Record name | 1,1′-Oxybis[4-methylbenzene] | |
Source | CAS Common Chemistry | |
URL | https://commonchemistry.cas.org/detail?cas_rn=1579-40-4 | |
Description | CAS Common Chemistry is an open community resource for accessing chemical information. Nearly 500,000 chemical substances from CAS REGISTRY cover areas of community interest, including common and frequently regulated chemicals, and those relevant to high school and undergraduate chemistry classes. This chemical information, curated by our expert scientists, is provided in alignment with our mission as a division of the American Chemical Society. | |
Explanation | The data from CAS Common Chemistry is provided under a CC-BY-NC 4.0 license, unless otherwise stated. | |
Record name | Benzene, 1,1'-oxybis(4-methyl- | |
Source | ChemIDplus | |
URL | https://pubchem.ncbi.nlm.nih.gov/substance/?source=chemidplus&sourceid=0001579404 | |
Description | ChemIDplus is a free, web search system that provides access to the structure and nomenclature authority files used for the identification of chemical substances cited in National Library of Medicine (NLM) databases, including the TOXNET system. | |
Record name | Benzene, 1,1'-oxybis[4-methyl- | |
Source | EPA Chemicals under the TSCA | |
URL | https://www.epa.gov/chemicals-under-tsca | |
Description | EPA Chemicals under the Toxic Substances Control Act (TSCA) collection contains information on chemicals and their regulations under TSCA, including non-confidential content from the TSCA Chemical Substance Inventory and Chemical Data Reporting. | |
Record name | p-Tolyl ether | |
Source | EPA DSSTox | |
URL | https://comptox.epa.gov/dashboard/DTXSID2061800 | |
Description | DSSTox provides a high quality public chemistry resource for supporting improved predictive toxicology. | |
Record name | Di-p-tolyl ether | |
Source | European Chemicals Agency (ECHA) | |
URL | https://echa.europa.eu/substance-information/-/substanceinfo/100.014.931 | |
Description | The European Chemicals Agency (ECHA) is an agency of the European Union which is the driving force among regulatory authorities in implementing the EU's groundbreaking chemicals legislation for the benefit of human health and the environment as well as for innovation and competitiveness. | |
Explanation | Use of the information, documents and data from the ECHA website is subject to the terms and conditions of this Legal Notice, and subject to other binding limitations provided for under applicable law, the information, documents and data made available on the ECHA website may be reproduced, distributed and/or used, totally or in part, for non-commercial purposes provided that ECHA is acknowledged as the source: "Source: European Chemicals Agency, http://echa.europa.eu/". Such acknowledgement must be included in each copy of the material. ECHA permits and encourages organisations and individuals to create links to the ECHA website under the following cumulative conditions: Links can only be made to webpages that provide a link to the Legal Notice page. | |
Synthesis routes and methods I
Procedure details
Synthesis routes and methods II
Procedure details
Avertissement et informations sur les produits de recherche in vitro
Veuillez noter que tous les articles et informations sur les produits présentés sur BenchChem sont destinés uniquement à des fins informatives. Les produits disponibles à l'achat sur BenchChem sont spécifiquement conçus pour des études in vitro, qui sont réalisées en dehors des organismes vivants. Les études in vitro, dérivées du terme latin "in verre", impliquent des expériences réalisées dans des environnements de laboratoire contrôlés à l'aide de cellules ou de tissus. Il est important de noter que ces produits ne sont pas classés comme médicaments et n'ont pas reçu l'approbation de la FDA pour la prévention, le traitement ou la guérison de toute condition médicale, affection ou maladie. Nous devons souligner que toute forme d'introduction corporelle de ces produits chez les humains ou les animaux est strictement interdite par la loi. Il est essentiel de respecter ces directives pour assurer la conformité aux normes légales et éthiques en matière de recherche et d'expérimentation.