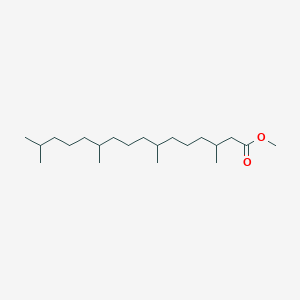
Hexadecanoic acid, 3,7,11,15-tetramethyl-, methyl ester
Vue d'ensemble
Description
Phytanic acid methyl ester, also known as 3,7,11,15-tetramethylhexadecanoic acid methyl ester, is a long-chain fatty acid methyl ester. It is an esterified form of phytanic acid, which is a bioactive multi-branched fatty acid. Phytanic acid is mainly synthesized by ruminants from trans-phytol, a compound found esterified in chlorophyll, the principal green pigment of plants .
Applications De Recherche Scientifique
Phytanic acid methyl ester has various scientific research applications, including:
Chemistry: It is used as a standard in gas chromatography and nuclear magnetic resonance (NMR) spectroscopy for the analysis of complex mixtures.
Biology: It is studied for its role in metabolic pathways and its effects on cellular processes.
Medicine: Research is conducted on its potential therapeutic effects and its role in metabolic disorders.
Industry: It is used in the production of biofuels and as a chemical intermediate in the synthesis of other compounds
Mécanisme D'action
Phytanic acid methyl ester exerts its effects through various molecular targets and pathways. It is involved in the protonophoric action, where the protonated form of fatty acids penetrates the membrane, and the ADP/ATP-translocase and other integral membrane proteins back-transport their anionic form. This mechanism is crucial in understanding its biological effects and potential therapeutic applications .
Analyse Biochimique
Biochemical Properties
Phytanic acid methyl ester plays a significant role in biochemical reactions, particularly in lipid metabolism. It interacts with several enzymes and proteins, including those involved in the α-oxidation pathway. One of the key enzymes it interacts with is phytanoyl-CoA hydroxylase, which catalyzes the first step in the α-oxidation of phytanic acid. This interaction is crucial for the conversion of phytanic acid to pristanic acid, which can then undergo β-oxidation .
Cellular Effects
Phytanic acid methyl ester influences various cellular processes, including cell signaling pathways, gene expression, and cellular metabolism. It has been shown to activate peroxisome proliferator-activated receptor alpha (PPAR-α) and retinoid X receptor (RXR), which are transcription factors involved in lipid metabolism and energy homeostasis. This activation can lead to changes in gene expression that promote fatty acid oxidation and reduce lipid accumulation in cells .
Molecular Mechanism
At the molecular level, phytanic acid methyl ester exerts its effects through binding interactions with specific biomolecules. It binds to PPAR-α and RXR, leading to the formation of heterodimers that regulate the transcription of target genes involved in lipid metabolism. Additionally, it can inhibit the activity of certain enzymes, such as acyl-CoA oxidase, which is involved in the β-oxidation of fatty acids .
Temporal Effects in Laboratory Settings
In laboratory settings, the effects of phytanic acid methyl ester can change over time due to its stability and degradation. The compound is relatively stable under standard storage conditions, but it can degrade over time, leading to a decrease in its efficacy. Long-term studies have shown that prolonged exposure to phytanic acid methyl ester can result in changes in cellular function, including alterations in lipid metabolism and energy homeostasis .
Dosage Effects in Animal Models
The effects of phytanic acid methyl ester vary with different dosages in animal models. At low doses, it can promote fatty acid oxidation and improve lipid metabolism. At high doses, it can have toxic effects, including liver damage and disruption of metabolic processes. Threshold effects have been observed, where the beneficial effects are seen at lower doses, while adverse effects occur at higher doses .
Metabolic Pathways
Phytanic acid methyl ester is involved in several metabolic pathways, including the α-oxidation and β-oxidation of fatty acids. It interacts with enzymes such as phytanoyl-CoA hydroxylase and acyl-CoA oxidase, which are crucial for the metabolism of branched-chain fatty acids. These interactions can affect metabolic flux and alter the levels of metabolites involved in lipid metabolism .
Transport and Distribution
Within cells and tissues, phytanic acid methyl ester is transported and distributed through interactions with specific transporters and binding proteins. It can be taken up by cells via fatty acid transport proteins and is distributed to various cellular compartments, including the mitochondria and peroxisomes, where it undergoes metabolic processing .
Subcellular Localization
Phytanic acid methyl ester is localized in specific subcellular compartments, including the peroxisomes and mitochondria. Its activity and function are influenced by its localization, as it undergoes α-oxidation in the peroxisomes and β-oxidation in the mitochondria. Targeting signals and post-translational modifications play a role in directing the compound to these specific compartments .
Méthodes De Préparation
Phytanic acid methyl ester can be synthesized from trans-phytol, which is released from chlorophyll by microorganisms. The synthetic route involves the isolation of trans-phytol from natural sources, such as fresh grass, followed by its conversion into phytanic acid. The phytanic acid is then esterified to form phytanic acid methyl ester .
Industrial production methods typically involve the use of high-speed counter-current chromatography (HSCCC) to isolate trans-phytol from natural sources. The trans-phytol is then converted into phytanic acid, which is subsequently esterified to form phytanic acid methyl ester .
Analyse Des Réactions Chimiques
Phytanic acid methyl ester undergoes various chemical reactions, including:
Oxidation: This reaction involves the addition of oxygen to the compound, often resulting in the formation of carboxylic acids.
Reduction: This reaction involves the removal of oxygen or the addition of hydrogen, leading to the formation of alcohols.
Substitution: This reaction involves the replacement of one functional group with another, such as the replacement of the ester group with a different functional group.
Common reagents and conditions used in these reactions include oxidizing agents like potassium permanganate for oxidation, reducing agents like lithium aluminum hydride for reduction, and nucleophiles for substitution reactions .
Comparaison Avec Des Composés Similaires
Phytanic acid methyl ester can be compared with other similar compounds, such as:
Methyl palmitate: Another long-chain fatty acid methyl ester, but with a straight-chain structure.
Methyl stearate: Similar to methyl palmitate but with a longer carbon chain.
Methyl oleate: An unsaturated fatty acid methyl ester with a double bond in its structure.
Phytanic acid methyl ester is unique due to its multi-branched structure, which distinguishes it from the straight-chain or unsaturated fatty acid methyl esters .
Propriétés
IUPAC Name |
methyl 3,7,11,15-tetramethylhexadecanoate | |
---|---|---|
Source | PubChem | |
URL | https://pubchem.ncbi.nlm.nih.gov | |
Description | Data deposited in or computed by PubChem | |
InChI |
InChI=1S/C21H42O2/c1-17(2)10-7-11-18(3)12-8-13-19(4)14-9-15-20(5)16-21(22)23-6/h17-20H,7-16H2,1-6H3 | |
Source | PubChem | |
URL | https://pubchem.ncbi.nlm.nih.gov | |
Description | Data deposited in or computed by PubChem | |
InChI Key |
LAWJUFPULQZGLF-UHFFFAOYSA-N | |
Source | PubChem | |
URL | https://pubchem.ncbi.nlm.nih.gov | |
Description | Data deposited in or computed by PubChem | |
Canonical SMILES |
CC(C)CCCC(C)CCCC(C)CCCC(C)CC(=O)OC | |
Source | PubChem | |
URL | https://pubchem.ncbi.nlm.nih.gov | |
Description | Data deposited in or computed by PubChem | |
Molecular Formula |
C21H42O2 | |
Source | PubChem | |
URL | https://pubchem.ncbi.nlm.nih.gov | |
Description | Data deposited in or computed by PubChem | |
DSSTOX Substance ID |
DTXSID50340862 | |
Record name | Hexadecanoic acid, 3,7,11,15-tetramethyl-, methyl ester | |
Source | EPA DSSTox | |
URL | https://comptox.epa.gov/dashboard/DTXSID50340862 | |
Description | DSSTox provides a high quality public chemistry resource for supporting improved predictive toxicology. | |
Molecular Weight |
326.6 g/mol | |
Source | PubChem | |
URL | https://pubchem.ncbi.nlm.nih.gov | |
Description | Data deposited in or computed by PubChem | |
CAS RN |
1118-77-0 | |
Record name | Hexadecanoic acid, 3,7,11,15-tetramethyl-, methyl ester | |
Source | EPA DSSTox | |
URL | https://comptox.epa.gov/dashboard/DTXSID50340862 | |
Description | DSSTox provides a high quality public chemistry resource for supporting improved predictive toxicology. | |
Record name | Phytanic acid methyl ester | |
Source | European Chemicals Agency (ECHA) | |
URL | https://echa.europa.eu/information-on-chemicals | |
Description | The European Chemicals Agency (ECHA) is an agency of the European Union which is the driving force among regulatory authorities in implementing the EU's groundbreaking chemicals legislation for the benefit of human health and the environment as well as for innovation and competitiveness. | |
Explanation | Use of the information, documents and data from the ECHA website is subject to the terms and conditions of this Legal Notice, and subject to other binding limitations provided for under applicable law, the information, documents and data made available on the ECHA website may be reproduced, distributed and/or used, totally or in part, for non-commercial purposes provided that ECHA is acknowledged as the source: "Source: European Chemicals Agency, http://echa.europa.eu/". Such acknowledgement must be included in each copy of the material. ECHA permits and encourages organisations and individuals to create links to the ECHA website under the following cumulative conditions: Links can only be made to webpages that provide a link to the Legal Notice page. | |
Retrosynthesis Analysis
AI-Powered Synthesis Planning: Our tool employs the Template_relevance Pistachio, Template_relevance Bkms_metabolic, Template_relevance Pistachio_ringbreaker, Template_relevance Reaxys, Template_relevance Reaxys_biocatalysis model, leveraging a vast database of chemical reactions to predict feasible synthetic routes.
One-Step Synthesis Focus: Specifically designed for one-step synthesis, it provides concise and direct routes for your target compounds, streamlining the synthesis process.
Accurate Predictions: Utilizing the extensive PISTACHIO, BKMS_METABOLIC, PISTACHIO_RINGBREAKER, REAXYS, REAXYS_BIOCATALYSIS database, our tool offers high-accuracy predictions, reflecting the latest in chemical research and data.
Strategy Settings
Precursor scoring | Relevance Heuristic |
---|---|
Min. plausibility | 0.01 |
Model | Template_relevance |
Template Set | Pistachio/Bkms_metabolic/Pistachio_ringbreaker/Reaxys/Reaxys_biocatalysis |
Top-N result to add to graph | 6 |
Feasible Synthetic Routes
Q & A
Q1: What are the common synthesis methods for Methyl Phytanate, and are there any involving labeled precursors?
A1: Methyl Phytanate can be synthesized from Phytol through a two-step process. First, Phytol undergoes catalytic hydrogenation. Then, the resulting product is oxidized using chromium oxide, yielding Methyl Phytanate. This method has been successfully employed to produce Methyl Phytanate with a carbon-14 label (Methyl Phytanate-(14)C) using a labeled precursor. []
Q2: Besides the traditional GC/MS, are there alternative analytical techniques suitable for characterizing Methyl Phytanate?
A2: While Gas Chromatography-Mass Spectrometry (GC/MS) remains a standard technique for analyzing Methyl Phytanate, Proton Nuclear Magnetic Resonance (1H-NMR) spectroscopy offers a complementary approach for structural characterization. This method provides detailed information about the hydrogen atoms within the molecule, offering insights into its structure and purity. []
Avertissement et informations sur les produits de recherche in vitro
Veuillez noter que tous les articles et informations sur les produits présentés sur BenchChem sont destinés uniquement à des fins informatives. Les produits disponibles à l'achat sur BenchChem sont spécifiquement conçus pour des études in vitro, qui sont réalisées en dehors des organismes vivants. Les études in vitro, dérivées du terme latin "in verre", impliquent des expériences réalisées dans des environnements de laboratoire contrôlés à l'aide de cellules ou de tissus. Il est important de noter que ces produits ne sont pas classés comme médicaments et n'ont pas reçu l'approbation de la FDA pour la prévention, le traitement ou la guérison de toute condition médicale, affection ou maladie. Nous devons souligner que toute forme d'introduction corporelle de ces produits chez les humains ou les animaux est strictement interdite par la loi. Il est essentiel de respecter ces directives pour assurer la conformité aux normes légales et éthiques en matière de recherche et d'expérimentation.