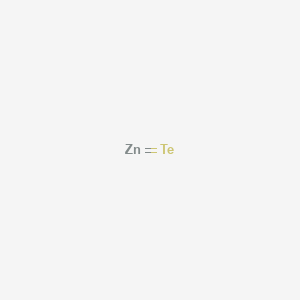
Zinc telluride
Vue d'ensemble
Description
Zinc telluride (ZnTe) is a II-VI compound semiconductor with a direct bandgap of approximately 2.26 eV at room temperature, making it suitable for optoelectronic applications such as light-emitting diodes, solar cells, and terahertz (THz) generation . It crystallizes in the cubic zincblende structure (space group F43m) and exhibits p-type conductivity, which distinguishes it from many other II-VI semiconductors . ZnTe thin films are synthesized via methods like electrodeposition, sputtering, and chemical vapor deposition, with post-annealing at ~683 K enhancing crystallinity and reducing resistivity from 2 × 10⁵ Ω·m (amorphous) to 2 × 10³ Ω·m (crystalline) . Its optical absorption edge at ~559 nm and compatibility with substrates like indium arsenide (InAs) further broaden its utility in heterojunction devices .
Applications De Recherche Scientifique
Photovoltaic Applications
Zinc Telluride in Solar Cells
this compound is primarily recognized for its role in thin-film solar cells, where it serves as an efficient light absorber. Its high absorption coefficient enables it to capture a substantial amount of solar energy, making it a promising candidate for next-generation solar technologies. Research efforts are focused on enhancing the performance of ZnTe-based solar cells to achieve higher energy conversion efficiencies.
Case Study: ZnTe Thin Films
A study demonstrated the effectiveness of ZnTe thin films in photovoltaic applications, highlighting their capability to form a liquid junction potential barrier when immersed in an electrolyte solution. This property facilitates the economic chemical route for trapping solar energy, thus improving the efficiency of photovoltaic cells .
Property | ZnTe |
---|---|
Band Gap | Wide (2.26 eV at room temp) |
Absorption Coefficient | High |
Application | Thin-film solar cells |
Optoelectronic Devices
Light-Emitting Diodes (LEDs) and Laser Diodes
ZnTe's direct bandgap allows for efficient light emission, making it suitable for manufacturing LEDs and laser diodes. The optoelectronic sector benefits from its unique properties, which contribute to improved performance and lower energy consumption in devices used for communication and display technologies.
Innovations in Optoelectronics
Recent advancements in ZnTe materials have led to better performance metrics in optoelectronic devices. For instance, researchers are exploring the integration of ZnTe into various optoelectronic systems to enhance their functionality and efficiency .
Telecommunications
Integration in Photonic Devices
this compound is becoming increasingly important in telecommunications, particularly in developing photonic devices that convert electrical signals into optical signals efficiently. Its application is vital for fiber optic communications, which require rapid data transfer capabilities.
Case Study: High-Speed Data Transfer
The integration of ZnTe into telecommunication infrastructure has shown promise in supporting high-speed data transfer requirements, reflecting the growing demand for advanced materials that facilitate faster and more reliable communication systems .
Environmental and Energy Applications
Photocatalysis and Water Splitting
Beyond electronics, this compound is being investigated for its potential environmental applications, particularly in photocatalysis for water splitting and pollutant degradation. Its ability to absorb sunlight makes it a candidate for sustainable energy solutions.
Research Findings
Studies indicate that ZnTe can effectively facilitate chemical reactions necessary for environmental remediation, showcasing its versatility beyond traditional semiconductor applications .
Market Trends and Future Outlook
The market for this compound is poised for growth as industries recognize its diverse applications across various sectors. Continuous research and development efforts aim to improve the material's properties while exploring new applications, particularly within renewable energy and optoelectronics.
Market Segment | Growth Potential |
---|---|
Solar Technology | High |
Optoelectronics | Moderate to High |
Telecommunications | Increasing Demand |
Environmental Tech | Emerging Opportunities |
Mécanisme D'action
The mechanism by which zinc telluride exerts its effects is primarily related to its semiconductor properties. When subjected to a high-intensity light pulse, this compound emits a pulse of terahertz frequency through a nonlinear optical process called optical rectification . This property makes it useful in various optoelectronic applications. Additionally, this compound’s ability to act as an electrocatalyst for water oxidation and nitrogen reduction is due to its favorable electronic properties and the efficient binding of reactant molecules to its surface .
Comparaison Avec Des Composés Similaires
Structural and Electronic Properties
Table 1: Key Properties of ZnTe and Comparable Semiconductors
Bandgap and Applications :
- Nonlinear Optical Performance: CdTe outperforms ZnTe in THz generation due to its higher nonlinear coefficient and lower bandgap, enabling efficient multiphoton absorption (MPA) at longer wavelengths .
Toxicity and Handling
- ZnTe reacts with acids to release toxic hydrogen telluride (H₂Te), akin to CdTe . However, Cd-containing compounds (CdTe, CZT) pose additional environmental risks due to cadmium’s bioaccumulation, necessitating stringent disposal protocols .
Commercial and Industrial Use
Optoelectronics :
ZnTe is used in tandem solar cells and quantum-well structures (e.g., CdₓZn₁₋ₓTe/ZnTe), leveraging its p-type conductivity for hole transport layers . CdTe dominates thin-film photovoltaics, while CZT is the gold standard for portable radiation detectors .Market Trends : The ZnTe market (CAS 1315-11-3) is niche compared to CdTe, with applications in research and specialized IR optics. Regional production is concentrated in Asia and Europe, with prices influenced by tellurium scarcity .
Activité Biologique
Zinc telluride (ZnTe) is a compound that has garnered attention in various fields, including electronics, optics, and increasingly, biomedicine. This article focuses on the biological activity of ZnTe, particularly its synthesis, characterization, and potential applications in biomedical fields such as drug delivery and antimicrobial agents.
Synthesis and Characterization
Recent studies have explored green synthesis methods for producing this compound nanoparticles (ZnTe QDs). One notable approach involves using plant extracts, such as those from Ficus johannis, to stabilize and reduce zinc and tellurium precursors into nanoparticles. This method not only simplifies the synthesis process but also enhances the biocompatibility of the nanoparticles.
Characterization Techniques
The synthesized ZnTe QDs are characterized using various techniques:
- X-ray Diffraction (XRD) : Confirms the crystalline nature of ZnTe.
- Transmission Electron Microscopy (TEM) : Reveals the morphology and size of nanoparticles (average size around 8 nm).
- Ultraviolet-Visible Absorption Spectroscopy : Indicates optical properties with a wide absorption band around 390 nm.
- Photoluminescence : Shows intense emission peaks at 463 nm, suggesting potential applications in optoelectronics.
Antioxidant Properties
ZnTe QDs exhibit significant antioxidant activity. Studies have demonstrated that these nanoparticles can scavenge free radicals effectively, which is crucial for mitigating oxidative stress in biological systems. The antioxidant capacity is often measured using assays like DPPH (2,2-diphenyl-1-picrylhydrazyl) and ABTS (2,2'-azino-bis(3-ethylbenzothiazoline-6-sulfonic acid)) assays.
Antimicrobial Activity
The antimicrobial properties of ZnTe QDs have been extensively studied. They show promising results against a range of pathogens:
- Bacteria : Effective against both Gram-positive (e.g., Staphylococcus aureus) and Gram-negative bacteria (e.g., Escherichia coli).
- Fungi : Demonstrated antifungal activity against species such as Candida albicans.
The mechanism of action is believed to involve the generation of reactive oxygen species (ROS) upon exposure to light or in contact with microbial cells, leading to cell membrane damage and ultimately cell death.
Case Studies
-
Antimicrobial Efficacy Study :
A study assessed the antibacterial activity of ZnTe QDs against E. coli and S. aureus. The results indicated a significant reduction in bacterial viability when exposed to varying concentrations of ZnTe QDs over a 24-hour period. The minimum inhibitory concentration (MIC) was determined to be around 50 µg/mL for both bacterial strains. -
Toxicity Assessment :
The toxicity of ZnTe QDs was evaluated using Artemia salina lethality tests. The results showed that at higher concentrations (>100 µg/mL), there was a notable increase in mortality rates among brine shrimp larvae, suggesting a dose-dependent toxicity profile.
Research Findings Summary
Analyse Des Réactions Chimiques
Chemical Bath Deposition (CBD)
CBD involves alkaline conditions with zinc sulfate (ZnSO₄), triethanolamine (TEA), ammonia (NH₃), and sodium telenosulfate (Na₂TeSO₃) at 369 K. Key steps:
- Complexation : Zn²⁺ forms a water-soluble complex with TEA, controlling free Zn²⁺ concentration.
- Hydrolysis : Na₂TeSO₃ dissociates to release Te²⁻ ions in alkaline media.
- Reaction :
Thickness increases linearly with deposition time, reaching 0.276 µm at 450 minutes .
Solid-State Reaction
ZnTe is prepared by heating stoichiometric Zn and Te in a vacuum-sealed quartz tube:
- Initial heating at 450°C for 24 hours.
- Temperature ramped to 850°C for homogenization (48 hours).
- Quenching in ice water yields polycrystalline ZnTe .
Electrochemical Reactions
ZnTe exhibits catalytic activity in nitrogen reduction and oxygen evolution:
Electrocatalytic Nitrogen Reduction (NRR)
ZnTe’s (111) plane preferentially adsorbs N₂ (adsorption energy = −0.043 eV) over H₂ (−0.028 eV), enabling ambient NH₃ synthesis:Density functional theory (DFT) confirms Te enhances electron transfer kinetics .
Oxygen Evolution Reaction (OER)
ZnTe nanospheres (ZnTe-NS) initiate OER at 1.41 V vs. RHE (η = 180 mV):Hydroxyl attachment energy on ZnTe-NS is −1.2 eV, ensuring durability (>50 hours) .
Electrodeposition
ZnTe films are electroplated via:
Reaction | Equation |
---|---|
Tellurium deposition | |
Zinc telluride formation | |
Optimal conditions: pH = 2.0, potential = −0.8 V vs. Ag/AgCl . |
Photoelectrochemical Reactions
ZnTe’s bandgap (2.26 eV) enables solar-driven applications:
Hydrogen Production
MoS₂/C-modified ZnTe photocathodes achieve 8.2 mA/cm² photocurrent at 0 V vs. RHE:Charge carrier lifetime exceeds 10 ns due to reduced recombination .
Photovoltaic Performance
ZnTe|polysulfide cells show:
Parameter | Value |
---|---|
Open-circuit voltage | 150 mV |
Short-circuit current | 25.6 µA |
Efficiency | 0.49% |
Barrier height = 0.583 eV, ideality factor = 2.87 . |
Reaction with Oxygen
ZnTe oxidizes exothermally under UV irradiation:XPS confirms Te⁴+ and Zn²+ oxidation states post-reaction .
Doping and Alloying
Selenium (Se) doping alters defect chemistry:
Doping Level | Conductivity Type | Bandgap (eV) |
---|---|---|
0% Se | p-type | 2.26 |
5% Se | n-type | 2.18 |
Se substitutes Te, reducing hole concentration by 10²⁰ cm⁻³ . |
Stability in Aqueous Media
Q & A
Basic Research Questions
Q. What are the primary methods for synthesizing high-purity Zinc Telluride crystals, and how do synthesis parameters influence crystal quality?
- Methodological Answer : ZnTe synthesis typically employs molecular beam epitaxy (MBE) or chemical vapor deposition (CVD) . Key parameters include:
- Substrate temperature (optimal range: 300–400°C for MBE ).
- Stoichiometric control of Zn:Te ratios to minimize defects like Te vacancies .
- Post-growth annealing in inert atmospheres (e.g., Ar) to enhance crystallinity .
- Characterization: Use X-ray diffraction (XRD) to confirm cubic zincblende structure and energy-dispersive X-ray spectroscopy (EDS) to verify stoichiometry .
Q. How do researchers characterize the structural and electronic properties of this compound thin films?
- Methodological Answer :
- Structural analysis :
- Atomic force microscopy (AFM) for surface roughness (<5 nm RMS for high-quality films ).
- Transmission electron microscopy (TEM) to identify grain boundaries and defects .
- Electronic properties :
- UV-Vis spectroscopy to measure the direct bandgap (~2.26 eV at 300K) .
- Hall effect measurements to determine carrier concentration and mobility (p-type conductivity with hole mobility ~40 cm²/V·s ).
Advanced Research Questions
Q. What experimental strategies resolve discrepancies in reported bandgap values of this compound under varying conditions?
- Methodological Answer : Contradictions in bandgap values (e.g., 2.2–2.4 eV) arise from doping effects , strain , or measurement techniques . To address this:
- Control synthesis conditions : Ensure identical substrate materials and growth rates to isolate strain effects .
- Cross-validate measurements : Combine ellipsometry , photoluminescence (PL) , and UV-Vis to account for instrumental biases .
- Statistical analysis : Use ANOVA to assess variability across batches .
Q. How can this compound-based detectors be optimized for high-resolution imaging in positron emission tomography (PET) systems?
- Methodological Answer : Key factors include:
- Crystal geometry : Use pixelated CdZnTe (CZT) detectors with <1 mm anode spacing to improve charge collection efficiency .
- Charge steering electrodes : Implement Frisch-grid designs to reduce signal noise and enhance spatial resolution (<0.5 mm FWHM ).
- Data acquisition : Optimize pulse-shaping algorithms to discriminate between gamma-ray interactions and background noise .
Q. What are the challenges in doping this compound with rare-earth elements (e.g., Gadolinium), and how do surface vs. bulk doping effects influence optoelectronic performance?
- Methodological Answer :
- Challenges : Rare-earth dopants (e.g., Gd³⁺) tend to segregate at grain boundaries, reducing doping efficiency .
- Surface vs. bulk effects :
- X-ray photoelectron spectroscopy (XPS) reveals surface oxidation (e.g., TeO₂ formation) that masks dopant signals .
- Secondary ion mass spectrometry (SIMS) quantifies bulk dopant distribution.
- Mitigation: Use encapsulation layers (e.g., Al₂O₃) during annealing to suppress surface oxidation .
Q. Data Contradiction and Reproducibility
Q. How should researchers address inconsistencies in phase diagram data for the Zn-Te system?
- Methodological Answer : Historical discrepancies (e.g., peritectic vs. eutectic reactions) stem from impurity levels and measurement techniques (e.g., differential thermal analysis vs. Knudsen cell vaporization ). To resolve:
- Reproduce experiments using ultra-high-purity precursors (>99.999%).
- Cross-reference with computational phase diagrams (CALPHAD models) .
Q. Experimental Design Tables
Table 1 : Synthesis Parameters for ZnTe Thin Films
Method | Temperature (°C) | Pressure (Torr) | Zn:Te Ratio | Bandgap (eV) | Reference |
---|---|---|---|---|---|
MBE | 320–380 | 10⁻⁸–10⁻¹⁰ | 1:1.05 | 2.24–2.28 | |
CVD | 450–500 | 10⁻²–10⁻³ | 1:1.1 | 2.20–2.25 |
Table 2 : Common Contradictions in ZnTe Research
Parameter | Reported Range | Likely Cause | Resolution Strategy |
---|---|---|---|
Bandgap (300K) | 2.2–2.4 eV | Strain, doping, measurement | Cross-validate with PL/UV-Vis |
Hole Mobility | 20–80 cm²/V·s | Defect density variations | Optimize annealing conditions |
Thermal Stability | Decomposition at 500°C vs. 600°C | Impurity-driven reactions | Use high-purity precursors |
Q. Key Recommendations
- Reproducibility : Document synthesis parameters (e.g., substrate type, gas flow rates) in line with Materials and Methods standards .
- Data Validation : Use open-access datasets (e.g., crystallography databases) to benchmark results .
- Ethical Practices : Cite primary literature and avoid over-reliance on secondary summaries .
Propriétés
IUPAC Name |
tellanylidenezinc | |
---|---|---|
Source | PubChem | |
URL | https://pubchem.ncbi.nlm.nih.gov | |
Description | Data deposited in or computed by PubChem | |
InChI |
InChI=1S/Te.Zn | |
Source | PubChem | |
URL | https://pubchem.ncbi.nlm.nih.gov | |
Description | Data deposited in or computed by PubChem | |
InChI Key |
NSRBDSZKIKAZHT-UHFFFAOYSA-N | |
Source | PubChem | |
URL | https://pubchem.ncbi.nlm.nih.gov | |
Description | Data deposited in or computed by PubChem | |
Canonical SMILES |
[Zn]=[Te] | |
Source | PubChem | |
URL | https://pubchem.ncbi.nlm.nih.gov | |
Description | Data deposited in or computed by PubChem | |
Molecular Formula |
ZnTe, TeZn | |
Record name | zinc telluride | |
Source | Wikipedia | |
URL | https://en.wikipedia.org/wiki/Zinc_telluride | |
Description | Chemical information link to Wikipedia. | |
Source | PubChem | |
URL | https://pubchem.ncbi.nlm.nih.gov | |
Description | Data deposited in or computed by PubChem | |
DSSTOX Substance ID |
DTXSID0061664 | |
Record name | Zinc telluride (ZnTe) | |
Source | EPA DSSTox | |
URL | https://comptox.epa.gov/dashboard/DTXSID0061664 | |
Description | DSSTox provides a high quality public chemistry resource for supporting improved predictive toxicology. | |
Molecular Weight |
193.0 g/mol | |
Source | PubChem | |
URL | https://pubchem.ncbi.nlm.nih.gov | |
Description | Data deposited in or computed by PubChem | |
Physical Description |
Grey or brownish-red solid; [Merck Index] Red lumps; [MSDSonline] | |
Record name | Zinc telluride | |
Source | Haz-Map, Information on Hazardous Chemicals and Occupational Diseases | |
URL | https://haz-map.com/Agents/9331 | |
Description | Haz-Map® is an occupational health database designed for health and safety professionals and for consumers seeking information about the adverse effects of workplace exposures to chemical and biological agents. | |
Explanation | Copyright (c) 2022 Haz-Map(R). All rights reserved. Unless otherwise indicated, all materials from Haz-Map are copyrighted by Haz-Map(R). No part of these materials, either text or image may be used for any purpose other than for personal use. Therefore, reproduction, modification, storage in a retrieval system or retransmission, in any form or by any means, electronic, mechanical or otherwise, for reasons other than personal use, is strictly prohibited without prior written permission. | |
CAS No. |
1315-11-3 | |
Record name | Zinc telluride | |
Source | CAS Common Chemistry | |
URL | https://commonchemistry.cas.org/detail?cas_rn=1315-11-3 | |
Description | CAS Common Chemistry is an open community resource for accessing chemical information. Nearly 500,000 chemical substances from CAS REGISTRY cover areas of community interest, including common and frequently regulated chemicals, and those relevant to high school and undergraduate chemistry classes. This chemical information, curated by our expert scientists, is provided in alignment with our mission as a division of the American Chemical Society. | |
Explanation | The data from CAS Common Chemistry is provided under a CC-BY-NC 4.0 license, unless otherwise stated. | |
Record name | Zinc telluride | |
Source | ChemIDplus | |
URL | https://pubchem.ncbi.nlm.nih.gov/substance/?source=chemidplus&sourceid=0001315113 | |
Description | ChemIDplus is a free, web search system that provides access to the structure and nomenclature authority files used for the identification of chemical substances cited in National Library of Medicine (NLM) databases, including the TOXNET system. | |
Record name | Zinc telluride (ZnTe) | |
Source | EPA Chemicals under the TSCA | |
URL | https://www.epa.gov/chemicals-under-tsca | |
Description | EPA Chemicals under the Toxic Substances Control Act (TSCA) collection contains information on chemicals and their regulations under TSCA, including non-confidential content from the TSCA Chemical Substance Inventory and Chemical Data Reporting. | |
Record name | Zinc telluride (ZnTe) | |
Source | EPA DSSTox | |
URL | https://comptox.epa.gov/dashboard/DTXSID0061664 | |
Description | DSSTox provides a high quality public chemistry resource for supporting improved predictive toxicology. | |
Record name | Zinc telluride | |
Source | European Chemicals Agency (ECHA) | |
URL | https://echa.europa.eu/substance-information/-/substanceinfo/100.013.874 | |
Description | The European Chemicals Agency (ECHA) is an agency of the European Union which is the driving force among regulatory authorities in implementing the EU's groundbreaking chemicals legislation for the benefit of human health and the environment as well as for innovation and competitiveness. | |
Explanation | Use of the information, documents and data from the ECHA website is subject to the terms and conditions of this Legal Notice, and subject to other binding limitations provided for under applicable law, the information, documents and data made available on the ECHA website may be reproduced, distributed and/or used, totally or in part, for non-commercial purposes provided that ECHA is acknowledged as the source: "Source: European Chemicals Agency, http://echa.europa.eu/". Such acknowledgement must be included in each copy of the material. ECHA permits and encourages organisations and individuals to create links to the ECHA website under the following cumulative conditions: Links can only be made to webpages that provide a link to the Legal Notice page. | |
Avertissement et informations sur les produits de recherche in vitro
Veuillez noter que tous les articles et informations sur les produits présentés sur BenchChem sont destinés uniquement à des fins informatives. Les produits disponibles à l'achat sur BenchChem sont spécifiquement conçus pour des études in vitro, qui sont réalisées en dehors des organismes vivants. Les études in vitro, dérivées du terme latin "in verre", impliquent des expériences réalisées dans des environnements de laboratoire contrôlés à l'aide de cellules ou de tissus. Il est important de noter que ces produits ne sont pas classés comme médicaments et n'ont pas reçu l'approbation de la FDA pour la prévention, le traitement ou la guérison de toute condition médicale, affection ou maladie. Nous devons souligner que toute forme d'introduction corporelle de ces produits chez les humains ou les animaux est strictement interdite par la loi. Il est essentiel de respecter ces directives pour assurer la conformité aux normes légales et éthiques en matière de recherche et d'expérimentation.