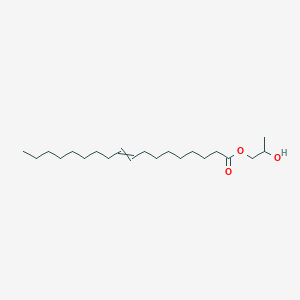
Propylene glycol monooleate
Vue d'ensemble
Description
Propylene glycol monooleate (PGMO) is a nonionic surfactant and emulsifier derived from propylene glycol and oleic acid. Its chemical structure consists of a propylene glycol backbone esterified with one oleic acid moiety. PGMO is widely utilized in pharmaceuticals, cosmetics, and food industries for its emulsifying, solubilizing, and stabilizing properties.
Méthodes De Préparation
Direct Esterification of Propylene Glycol and Oleic Acid
Direct esterification involves the reaction of propylene glycol with oleic acid under controlled conditions. This method is widely documented for its simplicity and scalability.
Chemical Catalysis
In conventional chemical catalysis, acidic or alkaline catalysts accelerate the dehydration reaction. For example, sulfuric acid or p-toluenesulfonic acid is used at elevated temperatures (150–200°C) to achieve esterification yields of 55–60% monoester content . However, excessive temperatures promote di-ester formation and side reactions, necessitating precise temperature control.
Enzymatic Catalysis
Enzymatic routes using lipases offer milder conditions and higher selectivity. Immobilized thermostable lipases, such as those from Pseudomonas species, enable reactions at 37–60°C with monoester yields exceeding 90% . A key advantage is the suppression of di-ester byproducts by maintaining water content below 0.5% . For instance, a packed-column system with immobilized lipase achieved 93.7% ester synthesis efficiency in 0.5–10 hours .
Table 1: Comparison of Direct Esterification Methods
Parameter | Chemical Catalysis | Enzymatic Catalysis |
---|---|---|
Temperature (°C) | 150–200 | 37–60 |
Reaction Time (h) | 4–8 | 0.5–10 |
Monoester Yield (%) | 55–60 | 90–93.7 |
Byproduct Formation | High | Low |
Sources |
Enzymatic Synthesis Optimization
Response surface methodology (RSM) has been employed to optimize enzymatic synthesis. A study using Candida antarctica lipase identified optimal conditions as 37.6°C, 7.6 hours, and a 2.6:1 molar ratio of lauric acid to propylene glycol, achieving 96% conversion . Ridge max analysis further confirmed these parameters, highlighting temperature and time as critical variables .
Solvent Systems
Non-aqueous solvents like tertiary alcohols (e.g., tert-pentanol) enhance lipase activity by stabilizing the enzyme’s open conformation. This approach reduces reaction times to 2–4 hours while maintaining yields above 90% .
Immobilization Techniques
Immobilizing lipases on silica or resin supports improves reusability. For example, lipase from Rhizomucor miehei retained 80% activity after 10 cycles in a batch reactor . Continuous-flow systems further enhance productivity, with monoester output rates of 15 g·L⁻¹·h⁻¹ reported .
Interesterification of Triglycerides
Interesterification involves reacting triglycerides (e.g., soybean oil) with propylene glycol to produce PGMO. This method is cost-effective due to the lower price of triglycerides compared to free fatty acids .
Reaction Conditions
Using alkaline catalysts like sodium hydroxide at 180–280°C, interesterification yields crude mixtures containing 65–75% monoesters . Oxygen-free environments (0–160 Torr) prevent oxidation, improving product color and stability .
Table 2: Interesterification Parameters and Outcomes
Catalyst | Temperature (°C) | Monoester Content (%) | Di-ester Content (%) |
---|---|---|---|
Sodium Hydroxide | 180–280 | 65–75 | 20–30 |
Potassium Acetate | 200–250 | 70–80 | 15–25 |
Sources |
Purification Techniques
Molecular distillation isolates monoesters from di-esters and glycerides. Vacuum distillation at 150–200°C and 0.1–1 mmHg achieves 95% monoester purity .
Solid Acid Catalysis
Acidic montmorillonite catalysts (e.g., KSF clay) enable esterification at 150°C with 93.5% oleic acid conversion . The catalyst’s Brønsted acidity facilitates protonation of the fatty acid, promoting nucleophilic attack by propylene glycol.
Molar Ratio Optimization
A 1:2 molar ratio of oleic acid to ethylene glycol maximizes conversion, while higher ratios (>1:3) increase viscosity and hinder mass transfer .
Catalyst Reusability
KSF clay retains 85% activity after five cycles, making it economically viable for continuous production .
Process Comparison and Industrial Scaling
Economic and Environmental Considerations
-
Enzymatic Methods : Lower energy consumption but higher enzyme costs.
-
Chemical Catalysis : Faster but generates more waste.
-
Interesterification : Most cost-effective for large-scale production .
Recent Advances
Novel biocatalysts (e.g., Thermomyces lanuginosus lipase) and microwave-assisted reactions reduce synthesis times to 30 minutes with 98% yields .
Analyse Des Réactions Chimiques
Propylene Oxide Addition to Oleic Acid
Reaction :
Transesterification of Triglycerides
Reaction :
-
Conditions :
-
Yield : ~55–60% monoester, with diester and unreacted triglycerides as byproducts .
-
Key Challenge : Requires oxygen-free conditions to prevent oxidation-induced discoloration .
Direct Esterification
Reaction :
Stability and Decomposition Reactions
PGMO undergoes degradation under specific conditions:
Thermal Decomposition
-
Pathway :
-
Conditions :
-
Impact : Color darkening (ΔE > 5) and rancid odor due to oxidation of unsaturated bonds .
Hydrolysis
Functionalization in Formulations
PGMO reacts with polar solvents to enhance drug delivery:
Solubilization with Propylene Glycol
-
Interaction : Forms reverse micelles in propylene glycol, increasing skin permeation of hydrophilic drugs (e.g., 5-aminolevulinic acid) .
-
Example :
Comparative Reaction Data
Applications De Recherche Scientifique
Food Industry
Propylene glycol monooleate is primarily used as an emulsifier in the food sector. Its properties enable it to stabilize mixtures of oil and water, making it essential in various food products.
Applications:
- Baking : It is commonly added to cake batters and shortenings to improve texture and shelf life. It helps maintain moisture and enhances the overall quality of baked goods .
- Dairy Products : Used in ice creams and margarine, it aids in achieving a smooth texture and preventing ice crystal formation .
- Sauces and Dressings : Acts as a stabilizer in emulsified sauces, ensuring uniform consistency .
Table 1: Food Applications of this compound
Application | Functionality |
---|---|
Baked Goods | Emulsifier for improved texture |
Ice Cream | Prevents ice crystal formation |
Sauces | Stabilizes emulsions |
Pharmaceuticals
In the pharmaceutical industry, this compound serves as a solvent and emulsifying agent in drug formulations.
Applications:
- Drug Delivery Systems : It enhances the solubility of hydrophobic drugs, facilitating their absorption in the body. This is particularly beneficial for oral and injectable medications .
- Topical Formulations : Used in creams and ointments, it helps to improve skin penetration of active ingredients .
Case Study:
A study demonstrated that formulations containing this compound significantly increased the skin permeation of 5-Aminolevulinic acid, a photosensitizer used in photodynamic therapy for skin cancer. The results indicated enhanced accumulation of protoporphyrin IX in mouse skin, suggesting improved efficacy of topical treatments .
Table 2: Pharmaceutical Applications of this compound
Application | Functionality |
---|---|
Drug Formulations | Solubilizes hydrophobic drugs |
Topical Creams | Enhances skin penetration |
Cosmetics
In cosmetics, this compound is utilized for its emulsifying properties, contributing to product stability and texture.
Applications:
- Lotions and Creams : It helps to blend oil and water components, providing a smooth application and enhancing moisturizing effects .
- Makeup Products : Used in foundations and other makeup items to improve spreadability and adherence to the skin .
Table 3: Cosmetic Applications of this compound
Application | Functionality |
---|---|
Lotions | Emulsifies oil and water |
Makeup Products | Enhances spreadability |
Industrial Applications
Beyond food, pharmaceuticals, and cosmetics, this compound finds use in industrial applications.
Applications:
Mécanisme D'action
The mechanism of action of propylene glycol monooleate involves its ability to interact with lipid bilayers and enhance the solubility and dispersion of other substances. It acts as a surfactant, reducing the surface tension between different phases, thereby stabilizing emulsions and dispersions. The compound can also penetrate biological membranes, enhancing the delivery of active ingredients in pharmaceutical and cosmetic formulations .
Comparaison Avec Des Composés Similaires
Key Identifiers :
- CAS Number : 107-98-2 .
- Applications : Emulsifier in topical formulations, stabilizer in food additives, and solubilizer in lipid-based drug delivery systems .
Structural and Functional Differences
The table below compares PGMO with structurally analogous compounds:
Performance in Surfactant Systems
- PGMO vs. Propylene Glycol Monolaurate: Propylene glycol monolaurate (PGML) demonstrates superior thickening in betaine and sulfate surfactant systems compared to coconut oil fatty acid monoethanolamide (CME). PGML also maintains foam stability and system clarity at low temperatures . While PGMO lacks direct thickening data, its oleate chain (C18:1) likely enhances lipophilicity, making it more suitable for oil-in-water emulsions.
- PGMO vs. Polyethylene Glycol Monooleate: Polyethylene glycol monooleate (PEG MO) has a lower molecular weight (326.51 vs. ~340) and higher water solubility due to its polyethylene glycol backbone. This grants PEG MO broader HLB versatility, whereas PGMO’s propylene glycol backbone favors lipid compatibility .
Regulatory and Industrial Relevance
- PGMO : Complies with USP standards for pharmaceutical use, particularly in topical gels .
- Propylene Glycol Monocaprylate: USP-referenced for solubilizing poorly water-soluble drugs .
- PEG MO : Technical-grade variants (~70% purity) are cost-effective for industrial emulsification .
Cosmetic and Detergent Performance
Activité Biologique
Propylene glycol monooleate (PGMO) is an emulsifier derived from the reaction of propylene glycol and oleic acid. It is widely used in food, pharmaceutical, and cosmetic formulations due to its surfactant properties. This article explores the biological activity of PGMO, focusing on its pharmacokinetics, metabolic pathways, and implications for health.
Pharmacokinetics
Absorption and Distribution
PGMO is rapidly absorbed after oral administration. Studies indicate that it reaches peak plasma concentrations within one hour. For example, Morshed et al. found that in rabbits, a dose of 2.942 g/kg resulted in a peak blood concentration of 41.04 mM after one hour . In humans, similar pharmacokinetic profiles have been observed, with a volume of distribution around 0.5 L/kg and a half-life ranging from 2.4 to 5.2 hours .
Metabolism
The primary metabolic pathway for PGMO involves oxidation by alcohol dehydrogenase to lactaldehyde, which is then converted to lactate by aldehyde dehydrogenase . Lactate can be further metabolized to pyruvate, carbon dioxide, and water, contributing to gluconeogenesis. Notably, the metabolic clearance rate differs between species; humans exhibit saturation at lower doses compared to rats and rabbits .
Biological Effects
Neurotoxicity
Research has indicated that PGMO may induce neurotoxic effects under certain conditions. A study on C57BL/6 mice demonstrated that exposure to PG at doses as low as 2 mL/kg led to significant apoptotic neurodegeneration in the developing central nervous system (CNS) . This raises concerns regarding its use in pediatric formulations where PG is commonly included as a solvent.
Skin Permeation Enhancement
PGMO has been shown to enhance the skin permeation of other compounds. For instance, in photodynamic therapy (PDT), formulations containing PGMO significantly improved the absorption of 5-aminolevulinic acid (5-ALA), a pro-drug for cancer treatment . This effect is attributed to its surfactant properties, which facilitate deeper penetration into skin layers.
Case Studies
Safety and Regulatory Status
PGMO is generally recognized as safe when used within regulated concentrations in food and pharmaceuticals. However, its potential neurotoxic effects necessitate careful consideration in pediatric applications . Regulatory bodies have evaluated its use extensively; it is included in various formulations across multiple industries.
Q & A
Basic Research Questions
Q. What are the validated methods for synthesizing propylene glycol monooleate, and how can reaction efficiency be optimized?
this compound is synthesized via esterification of oleic acid with propylene glycol. To optimize reaction efficiency, researchers should monitor molar ratios (e.g., 1:1 to 1:3 oleic acid to propylene glycol), use acid catalysts (e.g., sulfuric acid or enzymatic lipases), and control temperature (typically 80–120°C). Post-synthesis purification via vacuum distillation or molecular sieves removes unreacted glycol and fatty acids. Reaction progress can be tracked using acid value titration or FTIR to confirm ester bond formation .
Q. How can researchers characterize this compound’s purity and structural integrity?
Key methods include:
- Infrared Spectroscopy (FTIR) : To confirm ester carbonyl peaks (~1740 cm⁻¹) and hydroxyl groups .
- Gas Chromatography (GC) : Quantify residual ethylene oxide or dioxane contaminants (limits: ≤620 ppm for ethylene oxide) using N,N-dimethylacetamide extraction and sodium chloride partitioning .
- Fatty Acid Composition Analysis : Compare against pharmacopeial standards (e.g., USP) using GC-MS to verify oleic acid (C18:1) dominance (>90%) .
Q. What experimental protocols ensure accurate determination of hydrophilic-lipophilic balance (HLB) for formulation studies?
The HLB value (e.g., ~15.1 for this compound) is determined via Griffin’s method:
- Calculate using the formula: , where is the hydrophilic portion’s molecular weight.
- Validate experimentally by testing emulsification efficiency in oil-water systems (e.g., mineral oil/water). Adjust HLB by blending with low-HLB surfactants (e.g., Span 80) for stability .
Advanced Research Questions
Q. How do researchers resolve contradictions in stability data under varying storage conditions?
Stability studies should assess:
- Oxidative Degradation : Accelerated aging at 40°C/75% RH with peroxide value testing (limit: ≤6.0 mEq/kg) .
- Hydrolysis : Monitor free fatty acid content via acid value titration under acidic/basic conditions. Use nitrogen inerting during storage to mitigate oxidation .
- Compatibility : Screen for incompatibilities with amines, strong oxidizers, or aldehydes using differential scanning calorimetry (DSC) to detect exothermic reactions .
Q. What advanced analytical techniques are recommended for identifying degradation byproducts in pharmaceutical formulations?
- LC-MS/MS : Detect trace degradation products (e.g., mono- and diglycerides) with high sensitivity.
- NMR Spectroscopy : Resolve structural changes in the propylene glycol backbone or fatty acid chain .
- Accelerated Stability Testing : Use ICH Q1A guidelines (25°C/60% RH and 40°C/75% RH) to correlate degradation kinetics with Arrhenius models .
Q. How can researchers design experiments to evaluate this compound’s role in enhancing drug solubility?
- Phase Solubility Studies : Incrementally add the compound to a drug solution and measure solubility via UV-Vis or HPLC.
- Pseudoternary Phase Diagrams : Map microemulsion regions using water, oil (e.g., isopropyl myristate), and surfactant blends. Optimize ratios for maximal drug loading .
- In Vitro Permeation : Use Franz diffusion cells with synthetic membranes (e.g., Strat-M®) to assess bioavailability enhancement .
Q. Methodological Considerations Table
Research Focus | Key Parameters | Analytical Tools | Reference Standards |
---|---|---|---|
Synthesis Optimization | Molar ratio, catalyst type, temperature | FTIR, GC-MS | USP Propylene Glycol Monolaurate |
Stability Testing | Peroxide value, acid value, RH conditions | DSC, LC-MS/MS | PhEur Fatty Acid Composition |
Formulation Design | HLB, droplet size, drug loading | Dynamic Light Scattering, Phase Diagrams | FCC Polyethylene Glycol |
Propriétés
Numéro CAS |
1330-80-9 |
---|---|
Formule moléculaire |
C21H40O3 |
Poids moléculaire |
340.5 g/mol |
Nom IUPAC |
2-hydroxypropyl (E)-octadec-9-enoate |
InChI |
InChI=1S/C21H40O3/c1-3-4-5-6-7-8-9-10-11-12-13-14-15-16-17-18-21(23)24-19-20(2)22/h10-11,20,22H,3-9,12-19H2,1-2H3/b11-10+ |
Clé InChI |
ZVTDEEBSWIQAFJ-ZHACJKMWSA-N |
SMILES |
CCCCCCCCC=CCCCCCCCC(=O)OCC(C)O |
SMILES isomérique |
CCCCCCCC/C=C/CCCCCCCC(=O)OCC(C)O |
SMILES canonique |
CCCCCCCCC=CCCCCCCCC(=O)OCC(C)O |
Key on ui other cas no. |
1330-80-9 |
Description physique |
Liquid |
Origine du produit |
United States |
Retrosynthesis Analysis
AI-Powered Synthesis Planning: Our tool employs the Template_relevance Pistachio, Template_relevance Bkms_metabolic, Template_relevance Pistachio_ringbreaker, Template_relevance Reaxys, Template_relevance Reaxys_biocatalysis model, leveraging a vast database of chemical reactions to predict feasible synthetic routes.
One-Step Synthesis Focus: Specifically designed for one-step synthesis, it provides concise and direct routes for your target compounds, streamlining the synthesis process.
Accurate Predictions: Utilizing the extensive PISTACHIO, BKMS_METABOLIC, PISTACHIO_RINGBREAKER, REAXYS, REAXYS_BIOCATALYSIS database, our tool offers high-accuracy predictions, reflecting the latest in chemical research and data.
Strategy Settings
Precursor scoring | Relevance Heuristic |
---|---|
Min. plausibility | 0.01 |
Model | Template_relevance |
Template Set | Pistachio/Bkms_metabolic/Pistachio_ringbreaker/Reaxys/Reaxys_biocatalysis |
Top-N result to add to graph | 6 |
Feasible Synthetic Routes
Avertissement et informations sur les produits de recherche in vitro
Veuillez noter que tous les articles et informations sur les produits présentés sur BenchChem sont destinés uniquement à des fins informatives. Les produits disponibles à l'achat sur BenchChem sont spécifiquement conçus pour des études in vitro, qui sont réalisées en dehors des organismes vivants. Les études in vitro, dérivées du terme latin "in verre", impliquent des expériences réalisées dans des environnements de laboratoire contrôlés à l'aide de cellules ou de tissus. Il est important de noter que ces produits ne sont pas classés comme médicaments et n'ont pas reçu l'approbation de la FDA pour la prévention, le traitement ou la guérison de toute condition médicale, affection ou maladie. Nous devons souligner que toute forme d'introduction corporelle de ces produits chez les humains ou les animaux est strictement interdite par la loi. Il est essentiel de respecter ces directives pour assurer la conformité aux normes légales et éthiques en matière de recherche et d'expérimentation.