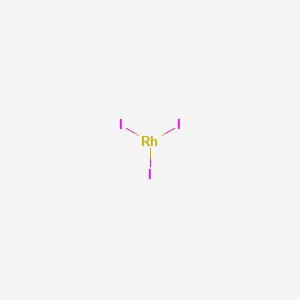
Rhodium triiodide
Vue d'ensemble
Description
Rhodium triiodide is a chemical compound with the formula RhI3. It is a dark purple solid that is insoluble in water. Rhodium triiodide has gained significant attention in the scientific community due to its potential applications in various fields, including catalysis, electronics, and materials science.
Mécanisme D'action
The mechanism of action of Rhodium triiodide is not fully understood. However, it is believed that the compound acts as a Lewis acid, which can accept electron pairs from other molecules. This property makes Rhodium triiodide an effective catalyst in organic reactions.
Biochemical and Physiological Effects:
There is limited research on the biochemical and physiological effects of Rhodium triiodide. However, studies have shown that the compound is relatively non-toxic and does not have any significant adverse effects on human health.
Avantages Et Limitations Des Expériences En Laboratoire
One of the main advantages of Rhodium triiodide is its effectiveness as a catalyst in organic reactions. However, the compound is relatively expensive and difficult to synthesize, which limits its use in some applications. Additionally, Rhodium triiodide is sensitive to air and moisture, which can affect its stability and reactivity.
Orientations Futures
There are several future directions for research on Rhodium triiodide. One area of interest is the development of new synthetic methods for the compound that are more cost-effective and scalable. Additionally, researchers are exploring the use of Rhodium triiodide in new applications, such as in the development of new materials for energy storage and conversion. Finally, there is ongoing research on the mechanism of action of Rhodium triiodide, which could lead to new insights into its catalytic properties and potential applications.
Méthodes De Synthèse
Rhodium triiodide can be synthesized by reacting rhodium metal with iodine in a sealed tube at high temperatures. The reaction produces a dark purple solid, which can be purified by sublimation.
Applications De Recherche Scientifique
Rhodium triiodide has been extensively studied for its potential applications in catalysis. It has been found to be an effective catalyst for various organic reactions, including the hydrogenation of alkenes and the dehydrogenation of alkanes. Rhodium triiodide has also been investigated for its potential use in electronics, particularly in the development of new materials for use in electronic devices.
Propriétés
IUPAC Name |
triiodorhodium | |
---|---|---|
Source | PubChem | |
URL | https://pubchem.ncbi.nlm.nih.gov | |
Description | Data deposited in or computed by PubChem | |
InChI |
InChI=1S/3HI.Rh/h3*1H;/q;;;+3/p-3 | |
Source | PubChem | |
URL | https://pubchem.ncbi.nlm.nih.gov | |
Description | Data deposited in or computed by PubChem | |
InChI Key |
KXAHUXSHRWNTOD-UHFFFAOYSA-K | |
Source | PubChem | |
URL | https://pubchem.ncbi.nlm.nih.gov | |
Description | Data deposited in or computed by PubChem | |
Canonical SMILES |
[Rh](I)(I)I | |
Source | PubChem | |
URL | https://pubchem.ncbi.nlm.nih.gov | |
Description | Data deposited in or computed by PubChem | |
Molecular Formula |
RhI3, I3Rh | |
Record name | Rhodium(III) iodide | |
Source | Wikipedia | |
URL | https://en.wikipedia.org/wiki/Rhodium(III)_iodide | |
Description | Chemical information link to Wikipedia. | |
Source | PubChem | |
URL | https://pubchem.ncbi.nlm.nih.gov | |
Description | Data deposited in or computed by PubChem | |
DSSTOX Substance ID |
DTXSID00329389 | |
Record name | Rhodium(III) iodide | |
Source | EPA DSSTox | |
URL | https://comptox.epa.gov/dashboard/DTXSID00329389 | |
Description | DSSTox provides a high quality public chemistry resource for supporting improved predictive toxicology. | |
Molecular Weight |
483.6189 g/mol | |
Source | PubChem | |
URL | https://pubchem.ncbi.nlm.nih.gov | |
Description | Data deposited in or computed by PubChem | |
Physical Description |
Black hygroscopic solid; [HSDB] Black hygroscopic crystals; Insoluble in water; [Alfa Aesar MSDS] | |
Record name | Rhodium iodide | |
Source | Haz-Map, Information on Hazardous Chemicals and Occupational Diseases | |
URL | https://haz-map.com/Agents/17103 | |
Description | Haz-Map® is an occupational health database designed for health and safety professionals and for consumers seeking information about the adverse effects of workplace exposures to chemical and biological agents. | |
Explanation | Copyright (c) 2022 Haz-Map(R). All rights reserved. Unless otherwise indicated, all materials from Haz-Map are copyrighted by Haz-Map(R). No part of these materials, either text or image may be used for any purpose other than for personal use. Therefore, reproduction, modification, storage in a retrieval system or retransmission, in any form or by any means, electronic, mechanical or otherwise, for reasons other than personal use, is strictly prohibited without prior written permission. | |
Product Name |
Rhodium triiodide | |
CAS RN |
15492-38-3 | |
Record name | Rhodium iodide (RhI3) | |
Source | CAS Common Chemistry | |
URL | https://commonchemistry.cas.org/detail?cas_rn=15492-38-3 | |
Description | CAS Common Chemistry is an open community resource for accessing chemical information. Nearly 500,000 chemical substances from CAS REGISTRY cover areas of community interest, including common and frequently regulated chemicals, and those relevant to high school and undergraduate chemistry classes. This chemical information, curated by our expert scientists, is provided in alignment with our mission as a division of the American Chemical Society. | |
Explanation | The data from CAS Common Chemistry is provided under a CC-BY-NC 4.0 license, unless otherwise stated. | |
Record name | Rhodium(III) iodide | |
Source | EPA DSSTox | |
URL | https://comptox.epa.gov/dashboard/DTXSID00329389 | |
Description | DSSTox provides a high quality public chemistry resource for supporting improved predictive toxicology. | |
Record name | Rhodium triiodide | |
Source | European Chemicals Agency (ECHA) | |
URL | https://echa.europa.eu/substance-information/-/substanceinfo/100.035.913 | |
Description | The European Chemicals Agency (ECHA) is an agency of the European Union which is the driving force among regulatory authorities in implementing the EU's groundbreaking chemicals legislation for the benefit of human health and the environment as well as for innovation and competitiveness. | |
Explanation | Use of the information, documents and data from the ECHA website is subject to the terms and conditions of this Legal Notice, and subject to other binding limitations provided for under applicable law, the information, documents and data made available on the ECHA website may be reproduced, distributed and/or used, totally or in part, for non-commercial purposes provided that ECHA is acknowledged as the source: "Source: European Chemicals Agency, http://echa.europa.eu/". Such acknowledgement must be included in each copy of the material. ECHA permits and encourages organisations and individuals to create links to the ECHA website under the following cumulative conditions: Links can only be made to webpages that provide a link to the Legal Notice page. | |
Avertissement et informations sur les produits de recherche in vitro
Veuillez noter que tous les articles et informations sur les produits présentés sur BenchChem sont destinés uniquement à des fins informatives. Les produits disponibles à l'achat sur BenchChem sont spécifiquement conçus pour des études in vitro, qui sont réalisées en dehors des organismes vivants. Les études in vitro, dérivées du terme latin "in verre", impliquent des expériences réalisées dans des environnements de laboratoire contrôlés à l'aide de cellules ou de tissus. Il est important de noter que ces produits ne sont pas classés comme médicaments et n'ont pas reçu l'approbation de la FDA pour la prévention, le traitement ou la guérison de toute condition médicale, affection ou maladie. Nous devons souligner que toute forme d'introduction corporelle de ces produits chez les humains ou les animaux est strictement interdite par la loi. Il est essentiel de respecter ces directives pour assurer la conformité aux normes légales et éthiques en matière de recherche et d'expérimentation.