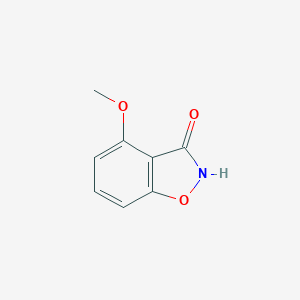
4-méthoxy-1,2-benzoxazol-3-one
- Cliquez sur DEMANDE RAPIDE pour recevoir un devis de notre équipe d'experts.
- Avec des produits de qualité à un prix COMPÉTITIF, vous pouvez vous concentrer davantage sur votre recherche.
Vue d'ensemble
Description
Synthesis Analysis
The synthesis of isoxazole derivatives, including compounds similar to 4-Methoxybenzo[d]isoxazol-3(2H)-one, often involves strategies such as 1,3-dipolar cycloadditions or modifications of pre-existing isoxazole frameworks. For example, Serebryannikova et al. (2019) reported the first synthesis of isoxazole-4-carboxylic acid derivatives by domino isoxazole-isoxazole isomerization, highlighting the versatility of methods available for constructing isoxazole-based compounds (Serebryannikova et al., 2019).
Molecular Structure Analysis
The molecular structure of 4-Methoxybenzo[d]isoxazol-3(2H)-one and its derivatives can be characterized using various spectroscopic techniques and theoretical calculations. Studies such as those by Kotan and Yuksek (2021) employ Density Functional Theory (DFT) to optimize the structure of related molecules, providing insights into their molecular geometry, vibrational frequencies, and electronic properties (Kotan & Yuksek, 2021).
Chemical Reactions and Properties
Isoxazole compounds, including 4-Methoxybenzo[d]isoxazol-3(2H)-one, participate in a variety of chemical reactions that highlight their reactivity and potential for further modification. The electrophilic reactivity of isoxazole derivatives has been demonstrated in studies like that of Cottyn et al. (2009), which explored the interaction of dinitrobenzo[d]isoxazole derivatives with methoxide ion, revealing the compound's versatility as an electrophile (Cottyn et al., 2009).
Applications De Recherche Scientifique
Activité antimicrobienne
Les dérivés de benzoxazole, y compris la 4-méthoxy-1,2-benzoxazol-3-one, ont été trouvés pour présenter une activité antimicrobienne significative. Ils ont été testés contre diverses souches bactériennes et fongiques, y compris une bactérie à Gram positif : Bacillus subtilis, quatre bactéries à Gram négatif : Escherichia coli, Pseudomonas aeruginosa, Klebsiella pneumoniae, Salmonella typhi et deux souches fongiques : Candida albicans et Aspergillus niger .
Activité anticancéreuse
Les composés benzoxazoliques ont également montré des résultats prometteurs dans les études anticancéreuses. Ils ont été testés contre la lignée cellulaire cancéreuse du carcinome colorectal humain (HCT116) et ont montré une activité anticancéreuse significative .
Sondes fluorescentes
Les dérivés de this compound présentent d'excellentes propriétés photophysiques comme de larges fenêtres spectrales, des valeurs d'absorptivité molaire élevées et une efficacité quantique de fluorescence raisonnablement bonne. Ces propriétés les rendent utiles comme sondes fluorescentes .
Intermédiaires pour les colorants
En raison de leurs excellentes propriétés photophysiques, les dérivés de this compound sont également utilisés comme intermédiaires pour les colorants .
Activité antifongique
Certains dérivés spécifiques de benzoxazole ont montré une activité antifongique puissante contre Aspergillus niger et Candida albicans .
Activité anti-inflammatoire
Il a été rapporté que les dérivés de benzoxazole présentent une activité anti-inflammatoire .
Activité antihistaminique
Il a également été rapporté que les dérivés de benzoxazole présentent une activité antihistaminique .
Activité antiparkinsonienne
Il a été rapporté que les dérivés de benzoxazole présentent une activité antiparkinsonienne .
Orientations Futures
Isoxazole derivatives, including “4-Methoxybenzo[d]isoxazol-3(2H)-one”, have potential applications in various fields, particularly in drug discovery due to their diverse biological activities . Future research could focus on exploring the therapeutic potential of these compounds, particularly their immunostimulatory properties .
Mécanisme D'action
Target of Action
The primary targets of 4-Methoxy-1,2-benzoxazol-3-one, a benzoxazole derivative, are key biological targets implicated in diseases such as cancer, diabetes, pain, inflammation, and cardiovascular disorders . The structural makeup of the benzoxazole scaffold allows efficient interaction with these biological targets .
Mode of Action
The planar benzene ring of the benzoxazole scaffold can form π-π stacking or π-cation interaction with the host molecule, whereas the 1-oxygen and 3-nitrogen of the oxazole moiety are hydrogen bond acceptors, hence, engage in non-covalent interactions . This interaction with its targets leads to changes in the biological activity of the targets, thereby affecting the disease state.
Biochemical Pathways
The benzoxazinoid biosynthetic pathway is involved in the synthesis of benzoxazole derivatives . This pathway involves nine enzymes that form a linear pathway leading to the storage of DI(M)BOA as glucoside conjugates . The first step of this pathway is catalyzed by an enzyme with indole-3-glycerol phosphate lyase activity .
Result of Action
Benzoxazole derivatives, including 4-Methoxy-1,2-benzoxazol-3-one, exhibit a wide range of biological activities including antimicrobial and anticancer properties. These compounds interact with their targets, leading to changes in cellular functions and potentially resulting in the death of cancer cells or inhibition of microbial growth.
Propriétés
IUPAC Name |
4-methoxy-1,2-benzoxazol-3-one |
Source
|
---|---|---|
Source | PubChem | |
URL | https://pubchem.ncbi.nlm.nih.gov | |
Description | Data deposited in or computed by PubChem | |
InChI |
InChI=1S/C8H7NO3/c1-11-5-3-2-4-6-7(5)8(10)9-12-6/h2-4H,1H3,(H,9,10) |
Source
|
Source | PubChem | |
URL | https://pubchem.ncbi.nlm.nih.gov | |
Description | Data deposited in or computed by PubChem | |
InChI Key |
LYGCVGSYMVVZKP-UHFFFAOYSA-N |
Source
|
Source | PubChem | |
URL | https://pubchem.ncbi.nlm.nih.gov | |
Description | Data deposited in or computed by PubChem | |
Canonical SMILES |
COC1=CC=CC2=C1C(=O)NO2 |
Source
|
Source | PubChem | |
URL | https://pubchem.ncbi.nlm.nih.gov | |
Description | Data deposited in or computed by PubChem | |
Molecular Formula |
C8H7NO3 |
Source
|
Source | PubChem | |
URL | https://pubchem.ncbi.nlm.nih.gov | |
Description | Data deposited in or computed by PubChem | |
Molecular Weight |
165.15 g/mol |
Source
|
Source | PubChem | |
URL | https://pubchem.ncbi.nlm.nih.gov | |
Description | Data deposited in or computed by PubChem | |
Avertissement et informations sur les produits de recherche in vitro
Veuillez noter que tous les articles et informations sur les produits présentés sur BenchChem sont destinés uniquement à des fins informatives. Les produits disponibles à l'achat sur BenchChem sont spécifiquement conçus pour des études in vitro, qui sont réalisées en dehors des organismes vivants. Les études in vitro, dérivées du terme latin "in verre", impliquent des expériences réalisées dans des environnements de laboratoire contrôlés à l'aide de cellules ou de tissus. Il est important de noter que ces produits ne sont pas classés comme médicaments et n'ont pas reçu l'approbation de la FDA pour la prévention, le traitement ou la guérison de toute condition médicale, affection ou maladie. Nous devons souligner que toute forme d'introduction corporelle de ces produits chez les humains ou les animaux est strictement interdite par la loi. Il est essentiel de respecter ces directives pour assurer la conformité aux normes légales et éthiques en matière de recherche et d'expérimentation.