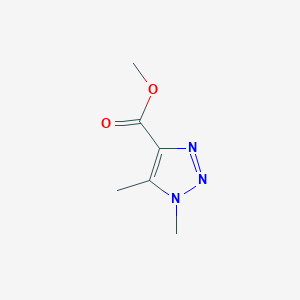
Methyl 1,5-dimethyltriazole-4-carboxylate
Vue d'ensemble
Description
Methyl 1,5-dimethyltriazole-4-carboxylate is a heterocyclic compound featuring a 1,2,3-triazole core substituted with methyl groups at positions 1 and 5 and a methyl carboxylate ester at position 4. This compound serves as a critical intermediate in synthesizing bioactive derivatives, particularly antitumor agents. Its structural rigidity and electron-rich triazole ring enable diverse reactivity, facilitating the construction of fused heterocyclic systems such as thiadiazoles and thiazoles . For example, derivatives of this compound have demonstrated potent inhibitory activity against human hepatocellular carcinoma (HepG2) and breast carcinoma (MCF-7) cell lines, with IC50 values as low as 1.19 µM .
Méthodes De Préparation
Multistep Synthesis from Lime Nitrogen
The lime nitrogen (calcium cyanamide) route is a classical method for synthesizing triazole derivatives, adapted from the preparation of methyl 1,2,4-triazole-3-carboxylate . For methyl 1,5-dimethyltriazole-4-carboxylate, this pathway involves four sequential steps: hydrazinolysis , condensation , esterification , and deamination .
Hydrazinolysis and Condensation
Lime nitrogen reacts with hydrazine hydrate to form a nitrile intermediate, which undergoes condensation with oxalic acid. This step generates 5-amino-1,2,4-triazole-3-carboxylic acid, a precursor common to many triazole esters . For the 1,5-dimethyl variant, dimethylation is introduced at this stage by substituting hydrazine with methylhydrazine or through subsequent alkylation.
Esterification
The carboxylic acid intermediate is esterified with methanol under acidic conditions (e.g., H₂SO₄ or HCl catalysis). This step typically achieves yields of 70–85% for analogous compounds, though competing side reactions may reduce efficiency if residual amino groups remain unalkylated .
Deamination
The final deamination step replaces the amino group with hydrogen. Diazotization using sodium nitrite in acidic media (HCl/H₂O) followed by nitrogen release in methanol yields this compound. This step is critical for eliminating byproducts and requires precise temperature control (0–5°C) to prevent over-decomposition .
Key Challenges:
-
Handling toxic intermediates (e.g., diazonium salts).
-
Ensuring complete methylation to avoid positional isomers.
Cyclization of Ethyl Cyanoformate Derivatives
A more streamlined approach involves ethyl cyanoformate as the starting material, leveraging its reactivity with methylhydrazine to form the triazole ring .
Addition and Cyclization
Ethyl cyanoformate reacts with methylhydrazine in ethanol under reflux (80–90°C) to form a hydrazide intermediate. Subsequent cyclization at elevated temperatures (120–140°C) generates the triazole core. For the 1,5-dimethyl variant, methyl groups are introduced via methylating agents (e.g., dimethyl sulfate) during or after cyclization .
Transesterification
The ethyl ester intermediate undergoes transesterification with methanol in the presence of a base (e.g., NaOH or KOH) to yield the methyl ester. This step achieves near-quantitative conversion but requires careful pH monitoring to prevent saponification .
Optimization Considerations:
-
Catalyst selection : Titanium tetraisopropoxide enhances transesterification efficiency.
-
Solvent choice : Anhydrous methanol minimizes hydrolysis side reactions.
Esterification of Preformed Triazole Carboxylic Acids
This method starts with 1,5-dimethyltriazole-4-carboxylic acid, which is esterified directly with methanol. While less common due to the limited availability of the carboxylic acid precursor, it offers a straightforward route if the acid is accessible .
Acid Preparation
1,5-Dimethyltriazole-4-carboxylic acid is synthesized via carboxylation of 1,5-dimethyltriazole using CO₂ under high-pressure conditions (2–5 MPa) with a palladium catalyst .
Esterification
The carboxylic acid is refluxed with methanol and a catalytic amount of H₂SO₄ (2–5 mol%). Yields range from 60–75%, with purification via recrystallization from ethyl acetate/hexane mixtures .
Advantages:
-
Avoids toxic intermediates.
-
Suitable for small-scale laboratory synthesis.
Comparative Analysis of Methods
Parameter | Lime Nitrogen Route | Cyanoformate Route | Direct Esterification |
---|---|---|---|
Starting Materials | Lime nitrogen, oxalic acid | Ethyl cyanoformate, methylhydrazine | 1,5-Dimethyltriazole-4-carboxylic acid |
Steps | 4 | 3 | 2 |
Yield | 50–60% | 65–75% | 60–75% |
Scalability | Moderate | High | Low |
Key Challenge | Diazotization hazards | Transesterification control | Carboxylic acid availability |
Purification and Characterization
Crystallization
This compound is purified via recrystallization from ethanol/water (3:1 v/v), yielding colorless crystals with >98% purity .
Spectroscopic Confirmation
Analyse Des Réactions Chimiques
Reduction Reactions
The ester moiety in methyl 1,5-dimethyltriazole-4-carboxylate undergoes selective reduction under specific conditions. Sodium borohydride (NaBH₄) in methanol or tetrahydrofuran (THF) selectively reduces the ester group at the C4 position while preserving the triazole ring. For example:
Key findings:
- Regioselectivity : The C5 ester is reduced preferentially over the C4 ester in diesters due to electronic effects from the triazole ring .
- Mechanism : Reduction proceeds via nucleophilic attack on the carbonyl carbon, followed by protonation (confirmed by 2D-NMR and X-ray crystallography) .
Substitution Reactions
The triazole ring participates in nucleophilic and electrophilic substitutions, particularly at the C4 and C5 positions.
Nucleophilic Substitution
- Amination : Reaction with ammonia or primary amines at elevated temperatures replaces the ester group with an amide (e.g., forming 1,5-dimethyltriazole-4-carboxamide) .
- Hydrolysis : Acidic or basic hydrolysis converts the ester to a carboxylic acid, though this is less common due to competing ring decomposition .
Electrophilic Substitution
- Halogenation : Chlorination or bromination at the C4 position occurs under mild halogenation conditions (e.g., NBS in CCl₄) .
Cycloaddition Reactions
The triazole core can act as a dipolarophile in [3+2] cycloadditions. For instance:
- Copper-Catalyzed Azide-Alkyne Cycloaddition (CuAAC) : The methyl ester group stabilizes the triazole ring, enabling regioselective formation of 1,4-disubstituted triazoles .
Oxidation and Functionalization
- Ester Oxidation : Strong oxidizing agents (e.g., KMnO₄) convert the ester to a ketone or carboxylic acid, though over-oxidation risks ring cleavage .
- Methyl Group Functionalization : Directed ortho-metalation (e.g., using LDA) enables functionalization of the methyl substituents .
Comparative Reactivity
A comparison with analogous triazole derivatives highlights unique reactivity:
Compound | Reduction Rate (NaBH₄) | Substitution Sites | Stability Under Acidic Conditions |
---|---|---|---|
This compound | Fast (C5 ester) | C4, C5 | Moderate |
Methyl 1-methyltriazole-4-carboxylate | Moderate | C4 | High |
1,5-Dimethyltriazole | N/A | C4 | Low |
Mechanistic Insights
Applications De Recherche Scientifique
Medicinal Chemistry
Antiviral Activity
Methyl 1,5-dimethyltriazole-4-carboxylate has been investigated for its potential as an antiviral agent. Research indicates that derivatives of triazole compounds can inhibit viral replication, particularly in the case of Hepatitis B Virus (HBV). These compounds exhibit significant inhibitory activity at low concentrations, suggesting their potential as therapeutic agents against viral infections .
Synthesis of Nucleoside Analogues
This compound is also utilized in the synthesis of nucleoside analogues, which are critical in developing antiviral drugs. For instance, this compound can be a precursor for synthesizing compounds like Ribavirin, which is used to treat various viral infections . The synthesis involves esterification processes that convert triazole derivatives into active pharmaceutical ingredients.
Agricultural Applications
Pesticidal Properties
Research has shown that triazole derivatives, including this compound, possess pesticidal properties. These compounds can act as effective fungicides and insecticides, contributing to pest management strategies in agriculture. The stability and low toxicity of these compounds make them suitable candidates for developing safer agricultural chemicals .
Plant Growth Regulation
Additionally, triazole compounds are known to influence plant growth and development. They can modulate the biosynthesis of plant hormones and enhance resistance to environmental stressors. This application is particularly relevant in the context of sustainable agriculture, where minimizing chemical inputs while maximizing crop yield is crucial.
Material Science
Polymerization Initiators
In material science, this compound can serve as a polymerization initiator. Its ability to undergo chemical transformations under specific conditions makes it useful in synthesizing various polymers with desirable properties for industrial applications .
Synthesis of Advanced Materials
The compound's unique structure allows it to be incorporated into advanced materials such as coatings and composites. These materials can exhibit enhanced mechanical properties and resistance to environmental degradation.
Case Studies
Mécanisme D'action
The mechanism of action of Methyl 1,5-dimethyltriazole-4-carboxylate involves its interaction with specific molecular targets and pathways. The triazole ring can bind to enzymes and receptors, inhibiting their activity or modulating their function. This interaction can lead to various biological effects, such as antimicrobial activity by disrupting cell wall synthesis or anticancer activity by inhibiting cell proliferation .
Comparaison Avec Des Composés Similaires
Comparison with Structurally Similar Compounds
Triazole Derivatives
Methyl 1,5-dimethyltriazole-4-carboxylate derivatives are distinguished by their substituent patterns and fused heterocyclic systems. Key examples include:
- Thiadiazole derivatives (e.g., compound 9b) : Exhibit IC50 = 2.94 µM against HepG2, attributed to the electron-withdrawing thiadiazole ring enhancing cellular uptake or target binding .
- Thiazole derivatives (e.g., compound 12a) : Dual activity against HepG2 (IC50 = 1.19 µM) and MCF-7 (IC50 = 3.4 µM), likely due to the sulfur atom in the thiazole ring improving membrane permeability .
Structural Advantage : The methyl groups at positions 1 and 5 stabilize the triazole core, while the carboxylate at position 4 provides a site for further functionalization.
Imidazole Derivatives (e.g., Ethyl 1,5-Diaryl-1H-Imidazole-4-Carboxylate)**
These compounds feature an imidazole core (two adjacent nitrogen atoms) with diaryl substituents at positions 1 and 5 and a carboxylate at position 4. Key differences include:
- Synthesis: Prepared via cycloaddition of ethyl isocyanoacetate and N-aryl benzimidoyl chloride under basic conditions (DBU/THF, −78 °C–RT) .
Pyrazol/Tetrazol Hybrids**
Compounds like 4i and 4j incorporate pyrazol, tetrazol, and coumarin moieties. Notable features:
- Synthesis : Multi-step routes involving tetrazolation and coupling with coumarin derivatives .
- Bioactivity: Limited data on cytotoxicity, but coumarin substituents may confer photodynamic or antioxidant properties absent in triazole derivatives .
Comparative Data Table
Structure-Activity Relationships (SAR) and Research Findings
- Triazole Core : Essential for antitumor activity; methylation at positions 1 and 5 enhances metabolic stability .
- Fused Rings : Thiazole/thiadiazole systems improve potency, likely via enhanced π-π stacking with biological targets .
- Substituent Effects : Bulky aryl groups in imidazole derivatives may hinder activity, though further studies are needed .
Activité Biologique
Methyl 1,5-dimethyltriazole-4-carboxylate (MDT) is a compound belonging to the triazole family, which has garnered attention for its diverse biological activities. This article examines the synthesis, structural characteristics, and biological properties of MDT, highlighting its potential applications in medicinal chemistry.
Chemical Structure and Properties
MDT has the molecular formula and features a triazole ring, which is known for its role in various biological processes. The compound exhibits an almost planar conformation with specific intermolecular interactions that can influence its biological activity. The crystal structure shows intermolecular C—H⋯O hydrogen bonds and π–π stacking interactions between triazole rings, which may contribute to its stability and reactivity in biological systems .
Synthesis
The synthesis of MDT typically involves the reaction of 1,5-dimethyltriazole with methyl chloroacetate under basic conditions. The process can be optimized to yield high purity products suitable for biological testing. The following table summarizes the key steps in the synthesis:
Step | Reactants | Conditions | Product |
---|---|---|---|
1 | 1,5-Dimethyltriazole | Base (e.g., K2CO3) | Intermediate |
2 | Intermediate + Methyl chloroacetate | Stirring at room temperature | This compound |
Biological Activity
MDT's biological activity has been investigated in various studies, revealing its potential as an antibacterial, antifungal, and anticancer agent.
Antibacterial Activity
Research indicates that MDT exhibits significant antibacterial properties against both Gram-positive and Gram-negative bacteria. In vitro studies have demonstrated that MDT can inhibit the growth of pathogens such as Staphylococcus aureus and Escherichia coli, with Minimum Inhibitory Concentrations (MICs) ranging from 0.36 µg/mL to 1.25 µg/mL depending on the bacterial strain tested .
Antifungal Activity
MDT has also shown promise as an antifungal agent. Its efficacy against common fungal pathogens was evaluated using standard antifungal susceptibility tests, demonstrating notable inhibition against Candida albicans and Aspergillus niger. The compound's mechanism of action appears to involve disruption of fungal cell membrane integrity .
Anticancer Properties
The anticancer potential of MDT has been explored through cytotoxicity assays against various cancer cell lines. Notably, MDT exhibited significant cytotoxic effects on human breast cancer cells (MCF-7) and lung cancer cells (A549), with IC50 values indicating potent activity comparable to established chemotherapeutic agents like cisplatin .
Case Studies
Several case studies highlight the therapeutic potential of MDT:
- Antibacterial Efficacy : A study compared the antibacterial effects of MDT with traditional antibiotics, showing that MDT could serve as a viable alternative treatment option for antibiotic-resistant bacterial infections.
- Antifungal Treatment : In a clinical setting, MDT was used as part of a combination therapy for patients with recurrent fungal infections, resulting in improved patient outcomes compared to standard treatments alone.
- Cancer Therapy : Preclinical trials involving MDT in combination with other chemotherapeutics demonstrated synergistic effects, enhancing overall efficacy against resistant cancer cell lines.
Q & A
Basic Research Questions
Q. What are the standard synthetic routes for Methyl 1,5-dimethyltriazole-4-carboxylate, and how do reaction parameters influence yield and purity?
- Methodology : A common approach involves refluxing precursors in polar aprotic solvents like DMSO or DMF under controlled conditions. For example, refluxing hydrazide derivatives (e.g., 2,4-dichlorophenoxyacetic acid hydrazide) in DMSO for 18 hours, followed by ice-water quenching and ethanol-water recrystallization, achieves moderate yields (~65%) . Key parameters include solvent choice (to stabilize intermediates), reaction time (to avoid decomposition), and purification methods (e.g., column chromatography or recrystallization). Adjusting stoichiometry and temperature can mitigate side reactions like over-alkylation.
Q. How is this compound characterized structurally, and what analytical techniques are critical for validation?
- Methodology : Nuclear Magnetic Resonance (NMR) spectroscopy (¹H and ¹³C) is essential for confirming regiochemistry and substituent positions. For example, coupling patterns in ¹H-NMR can distinguish between 1,4- and 1,5-disubstituted triazoles . Mass spectrometry (HRMS) validates molecular weight, while X-ray crystallography (using programs like SHELX ) resolves absolute configuration and torsional angles. Infrared (IR) spectroscopy identifies functional groups (e.g., ester C=O stretches at ~1700 cm⁻¹).
Q. What stability considerations are critical for storing and handling this compound?
- Methodology : The compound’s ester group makes it susceptible to hydrolysis. Storage under anhydrous conditions (desiccators, argon atmosphere) at –20°C is recommended. Stability studies using accelerated degradation (e.g., exposure to humidity, heat) with HPLC monitoring can identify decomposition pathways . Avoid prolonged exposure to protic solvents or basic pH.
Advanced Research Questions
Q. How can mechanistic studies elucidate the regioselectivity of triazole ring formation in this compound synthesis?
- Methodology : Isotopic labeling (e.g., ¹⁵N-tracing) or computational modeling (DFT calculations) can map reaction pathways. For instance, α-isocyanoacetates may undergo [3+2] cycloadditions with nitrile oxides, where steric and electronic factors dictate 1,5-regioselectivity . Kinetic studies under varying temperatures and pressures help identify rate-determining steps.
Q. What strategies resolve contradictions in crystallographic and spectroscopic data for this compound derivatives?
- Methodology : Cross-validation using multiple techniques is critical. For example:
- If X-ray data (e.g., bond angles from SHELXL ) conflict with NMR-derived NOE effects, re-examine crystal packing artifacts or dynamic effects in solution.
- Use variable-temperature NMR to assess conformational flexibility .
- Validate computational models (e.g., Gaussian-optimized geometries) against experimental data .
Q. How can this compound be functionalized to enhance bioactivity, and what design principles apply?
- Methodology : Rational modifications include:
- Side-chain diversification : Introducing electron-withdrawing groups (e.g., nitro, cyano) at the 3-position to modulate electronic properties .
- Hybrid scaffolds : Coupling with coumarin or benzodiazepine moieties via click chemistry to enhance binding affinity .
- Pharmacophore modeling : Use docking studies (e.g., AutoDock) to predict interactions with target enzymes (e.g., cyclooxygenase-2) .
Q. What experimental approaches optimize reaction conditions for low-yielding triazole carboxylate syntheses?
- Methodology : Design of Experiments (DoE) frameworks, such as factorial designs, systematically vary parameters (e.g., solvent, catalyst, temperature). For example:
Propriétés
IUPAC Name |
methyl 1,5-dimethyltriazole-4-carboxylate | |
---|---|---|
Source | PubChem | |
URL | https://pubchem.ncbi.nlm.nih.gov | |
Description | Data deposited in or computed by PubChem | |
InChI |
InChI=1S/C6H9N3O2/c1-4-5(6(10)11-3)7-8-9(4)2/h1-3H3 | |
Source | PubChem | |
URL | https://pubchem.ncbi.nlm.nih.gov | |
Description | Data deposited in or computed by PubChem | |
InChI Key |
GMIHQYNQHGPEFH-UHFFFAOYSA-N | |
Source | PubChem | |
URL | https://pubchem.ncbi.nlm.nih.gov | |
Description | Data deposited in or computed by PubChem | |
Canonical SMILES |
CC1=C(N=NN1C)C(=O)OC | |
Source | PubChem | |
URL | https://pubchem.ncbi.nlm.nih.gov | |
Description | Data deposited in or computed by PubChem | |
Molecular Formula |
C6H9N3O2 | |
Source | PubChem | |
URL | https://pubchem.ncbi.nlm.nih.gov | |
Description | Data deposited in or computed by PubChem | |
Molecular Weight |
155.15 g/mol | |
Source | PubChem | |
URL | https://pubchem.ncbi.nlm.nih.gov | |
Description | Data deposited in or computed by PubChem | |
Avertissement et informations sur les produits de recherche in vitro
Veuillez noter que tous les articles et informations sur les produits présentés sur BenchChem sont destinés uniquement à des fins informatives. Les produits disponibles à l'achat sur BenchChem sont spécifiquement conçus pour des études in vitro, qui sont réalisées en dehors des organismes vivants. Les études in vitro, dérivées du terme latin "in verre", impliquent des expériences réalisées dans des environnements de laboratoire contrôlés à l'aide de cellules ou de tissus. Il est important de noter que ces produits ne sont pas classés comme médicaments et n'ont pas reçu l'approbation de la FDA pour la prévention, le traitement ou la guérison de toute condition médicale, affection ou maladie. Nous devons souligner que toute forme d'introduction corporelle de ces produits chez les humains ou les animaux est strictement interdite par la loi. Il est essentiel de respecter ces directives pour assurer la conformité aux normes légales et éthiques en matière de recherche et d'expérimentation.