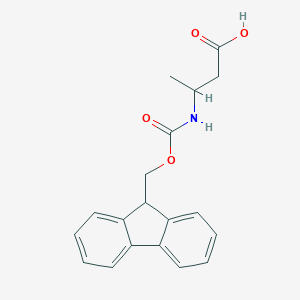
Acide Fmoc-DL-3-aminobutyrique
Vue d'ensemble
Description
3-((((9H-Fluoren-9-yl)methoxy)carbonyl)amino)butanoic acid is a useful research compound. Its molecular formula is C19H19NO4 and its molecular weight is 325.4 g/mol. The purity is usually 95%.
BenchChem offers high-quality 3-((((9H-Fluoren-9-yl)methoxy)carbonyl)amino)butanoic acid suitable for many research applications. Different packaging options are available to accommodate customers' requirements. Please inquire for more information about 3-((((9H-Fluoren-9-yl)methoxy)carbonyl)amino)butanoic acid including the price, delivery time, and more detailed information at info@benchchem.com.
Applications De Recherche Scientifique
Blocs de construction chimiques
L'acide Fmoc-DL-3-aminobutyrique est utilisé comme bloc de construction chimique dans la recherche en sciences de la vie . Il a une formule moléculaire de C19H19NO4 et un poids moléculaire de 325.36 .
Fabrication de matériaux fonctionnels
Les acides aminés modifiés par Fmoc, y compris l'this compound, sont des blocs de construction bio-inspirés pour la fabrication de matériaux fonctionnels . L'hydrophobie et l'aromaticité inhérentes du groupement Fmoc favorisent l'association des blocs de construction, ce qui les rend adaptés à diverses applications .
Caractéristiques d'auto-assemblage
Les acides aminés modifiés par Fmoc possèdent des caractéristiques d'auto-assemblage éminentes . Cette propriété est cruciale dans le domaine de la nanotechnologie, où les matériaux auto-assemblants peuvent constituer la base de la construction de nanostructures.
Systèmes de délivrance de médicaments
La caractéristique d'auto-assemblage des acides aminés modifiés par Fmoc en fait également des candidats potentiels pour les systèmes de délivrance de médicaments . La capacité à former des structures stables à l'échelle nanométrique peut être utilisée pour encapsuler les médicaments et les délivrer à des cibles spécifiques dans le corps.
Applications thérapeutiques
Les acides aminés modifiés par Fmoc présentent un potentiel distinct pour les applications thérapeutiques . La modification avec le groupement Fmoc peut améliorer la stabilité et la biodisponibilité des peptides thérapeutiques, améliorant ainsi leur efficacité.
Propriétés antibiotiques
Les acides aminés modifiés par Fmoc ont été étudiés pour leurs propriétés antibiotiques . La capacité à perturber les membranes cellulaires bactériennes en fait des candidats potentiels pour le développement de nouveaux antibiotiques.
Mécanisme D'action
- Specifically, the Fmoc group serves as a temporary protecting group for the N-terminal amino group, preventing unwanted reactions during peptide assembly .
- Piperidine forms a stable adduct with the dibenzofulvene byproduct, preventing it from reacting with the substrate .
Target of Action
Mode of Action
Biochemical Pathways
Analyse Biochimique
Biochemical Properties
It is known that the Fmoc group, which is part of the compound’s structure, has significant self-assembly features This property could potentially influence its interactions with enzymes, proteins, and other biomolecules
Cellular Effects
Given its structural similarity to other Fmoc-modified amino acids, it may have potential effects on cell function, including impacts on cell signaling pathways, gene expression, and cellular metabolism .
Molecular Mechanism
It is known that the Fmoc group can promote the association of building blocks due to its inherent hydrophobicity and aromaticity . This could potentially influence its binding interactions with biomolecules, enzyme inhibition or activation, and changes in gene expression.
Activité Biologique
3-((((9H-Fluoren-9-yl)methoxy)carbonyl)amino)butanoic acid, commonly referred to as Fmoc-Asp(OH)-OtBu, is a compound of significant interest in the fields of medicinal chemistry and biochemistry. This article explores its biological activity, including mechanisms of action, potential therapeutic applications, and relevant case studies.
- IUPAC Name : (R)-3-((((9H-fluoren-9-yl)methoxy)carbonyl)amino)-4-(3,5-difluorophenyl)butanoic acid
- Molecular Formula : C25H21F2NO4
- Molecular Weight : 437.44 g/mol
- CAS Number : 2349961-66-4
Structural Representation
The compound features a fluorenylmethoxy carbonyl group, which is significant for its interactions with biological targets.
Fmoc-Asp(OH)-OtBu exhibits biological activity primarily through its role as a peptide coupling agent. The fluorenylmethoxy carbonyl (Fmoc) group is widely utilized in solid-phase peptide synthesis, facilitating the formation of peptide bonds by protecting the amino group during synthesis.
Therapeutic Applications
Research indicates that this compound may have implications in:
- Anticancer Therapy : Preliminary studies suggest that derivatives of Fmoc-Asp(OH)-OtBu can inhibit tumor growth by interfering with cell signaling pathways associated with cancer progression.
- Neuroprotection : Some derivatives demonstrate neuroprotective effects, potentially useful in treating neurodegenerative diseases.
Case Studies and Research Findings
- Anticancer Activity :
- Neuroprotective Effects :
- Peptide Synthesis :
Data Table: Biological Activity Summary
Propriétés
IUPAC Name |
3-(9H-fluoren-9-ylmethoxycarbonylamino)butanoic acid | |
---|---|---|
Source | PubChem | |
URL | https://pubchem.ncbi.nlm.nih.gov | |
Description | Data deposited in or computed by PubChem | |
InChI |
InChI=1S/C19H19NO4/c1-12(10-18(21)22)20-19(23)24-11-17-15-8-4-2-6-13(15)14-7-3-5-9-16(14)17/h2-9,12,17H,10-11H2,1H3,(H,20,23)(H,21,22) | |
Source | PubChem | |
URL | https://pubchem.ncbi.nlm.nih.gov | |
Description | Data deposited in or computed by PubChem | |
InChI Key |
LYMLSPRRJWJJQD-UHFFFAOYSA-N | |
Source | PubChem | |
URL | https://pubchem.ncbi.nlm.nih.gov | |
Description | Data deposited in or computed by PubChem | |
Canonical SMILES |
CC(CC(=O)O)NC(=O)OCC1C2=CC=CC=C2C3=CC=CC=C13 | |
Source | PubChem | |
URL | https://pubchem.ncbi.nlm.nih.gov | |
Description | Data deposited in or computed by PubChem | |
Molecular Formula |
C19H19NO4 | |
Source | PubChem | |
URL | https://pubchem.ncbi.nlm.nih.gov | |
Description | Data deposited in or computed by PubChem | |
DSSTOX Substance ID |
DTXSID20407597 | |
Record name | 3-({[(9H-Fluoren-9-yl)methoxy]carbonyl}amino)butanoic acid | |
Source | EPA DSSTox | |
URL | https://comptox.epa.gov/dashboard/DTXSID20407597 | |
Description | DSSTox provides a high quality public chemistry resource for supporting improved predictive toxicology. | |
Molecular Weight |
325.4 g/mol | |
Source | PubChem | |
URL | https://pubchem.ncbi.nlm.nih.gov | |
Description | Data deposited in or computed by PubChem | |
CAS No. |
186320-18-3 | |
Record name | 3-({[(9H-Fluoren-9-yl)methoxy]carbonyl}amino)butanoic acid | |
Source | EPA DSSTox | |
URL | https://comptox.epa.gov/dashboard/DTXSID20407597 | |
Description | DSSTox provides a high quality public chemistry resource for supporting improved predictive toxicology. | |
Retrosynthesis Analysis
AI-Powered Synthesis Planning: Our tool employs the Template_relevance Pistachio, Template_relevance Bkms_metabolic, Template_relevance Pistachio_ringbreaker, Template_relevance Reaxys, Template_relevance Reaxys_biocatalysis model, leveraging a vast database of chemical reactions to predict feasible synthetic routes.
One-Step Synthesis Focus: Specifically designed for one-step synthesis, it provides concise and direct routes for your target compounds, streamlining the synthesis process.
Accurate Predictions: Utilizing the extensive PISTACHIO, BKMS_METABOLIC, PISTACHIO_RINGBREAKER, REAXYS, REAXYS_BIOCATALYSIS database, our tool offers high-accuracy predictions, reflecting the latest in chemical research and data.
Strategy Settings
Precursor scoring | Relevance Heuristic |
---|---|
Min. plausibility | 0.01 |
Model | Template_relevance |
Template Set | Pistachio/Bkms_metabolic/Pistachio_ringbreaker/Reaxys/Reaxys_biocatalysis |
Top-N result to add to graph | 6 |
Feasible Synthetic Routes
Avertissement et informations sur les produits de recherche in vitro
Veuillez noter que tous les articles et informations sur les produits présentés sur BenchChem sont destinés uniquement à des fins informatives. Les produits disponibles à l'achat sur BenchChem sont spécifiquement conçus pour des études in vitro, qui sont réalisées en dehors des organismes vivants. Les études in vitro, dérivées du terme latin "in verre", impliquent des expériences réalisées dans des environnements de laboratoire contrôlés à l'aide de cellules ou de tissus. Il est important de noter que ces produits ne sont pas classés comme médicaments et n'ont pas reçu l'approbation de la FDA pour la prévention, le traitement ou la guérison de toute condition médicale, affection ou maladie. Nous devons souligner que toute forme d'introduction corporelle de ces produits chez les humains ou les animaux est strictement interdite par la loi. Il est essentiel de respecter ces directives pour assurer la conformité aux normes légales et éthiques en matière de recherche et d'expérimentation.