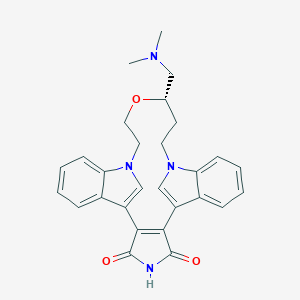
Ruboxistaurin
Vue d'ensemble
Description
Ruboxistaurin is an investigational drug that has been studied for the basic science of Type 2 Diabetes Mellitus and Type 1 Diabetes Mellitus . It is a small molecule and a member of the bisindolylmaleimide family .
Synthesis Analysis
The synthesis of Ruboxistaurin involves several steps, including the preparation of L-2-deoxyribose 1-cyano-3,4-dibenzoate, oxidative cleaving to (S)-4-hydroxy-2-(2-hydroxy-ethoxy)-butyronitrile, and transformation to the methanesulfonic acid (S)-3-cyano-3-(2-methanesulfonyloxyethoxy)-propyl ester .Molecular Structure Analysis
Ruboxistaurin has a molecular formula of C28H28N4O3 and an average molecular weight of 468.5469 . It is a member of the macrolactams, a class of organic compounds known as cyclic amides of amino carboxylic acids .Physical And Chemical Properties Analysis
Ruboxistaurin is a solid substance . It has a molecular weight of 468.55 . The storage conditions vary depending on whether it is in powder form or in solvent .Applications De Recherche Scientifique
Treatment of Diabetic Retinopathy
Ruboxistaurin (RBX) is an anti-vascular endothelial growth factor (anti-VEGF) agent that is used in the treatment of diabetic retinopathy . It is mainly given intravitreally . The drug is used to prevent vision loss in patients with diabetic retinopathy .
Nanoparticle Delivery System
RBX has been used in the development of a nanoparticle delivery system using polyamidoamine (PAMAM) dendrimers . This nanoformulation improves the therapeutic outcomes of anti-VEGF therapy and the bioavailability of RBX .
Treatment of Diabetic Macular Edema
RBX has been studied for its effects on diabetic macular edema (DME). It has been found to ameliorate the decrease in visual acuity associated with long-standing DME .
Protein Kinase C (PKC) Inhibition
Ruboxistaurin is a potent inhibitor of PKC, specifically the β1 and β2 isoforms . This inhibition is thought to be responsible for many of its therapeutic effects .
Treatment of Type 2 Diabetes Mellitus
Ruboxistaurin has been investigated for its potential use in the treatment of Type 2 Diabetes Mellitus .
Treatment of Type 1 Diabetes Mellitus
In addition to Type 2 Diabetes, Ruboxistaurin has also been studied for its potential use in the treatment of Type 1 Diabetes Mellitus .
Mécanisme D'action
Target of Action
Ruboxistaurin primarily targets Protein Kinase C-beta (PKC-β) . PKC-β is an enzyme that plays a crucial role in several signal transduction pathways, influencing cell proliferation, differentiation, and apoptosis .
Mode of Action
Ruboxistaurin acts as an inhibitor of PKC-β . By inhibiting PKC-β, ruboxistaurin can modulate the enzyme’s activity, leading to alterations in the signal transduction pathways that the enzyme is involved in .
Biochemical Pathways
Ruboxistaurin’s inhibition of PKC-β affects several biochemical pathways. One key pathway is the TGF-β1/Smad pathway , which is involved in cell proliferation and differentiation . Ruboxistaurin has been shown to down-regulate this pathway, thereby potentially exerting a renoprotective effect .
Pharmacokinetics
The pharmacokinetics of ruboxistaurin are linear within the studied dose range . Steady state is achieved after 7–21 days with twice-daily dosing . Ruboxistaurin given in the evening has lower plasma concentrations and a longer time to maximal concentration compared with the morning dosage .
Result of Action
The inhibition of PKC-β by ruboxistaurin has several effects at the molecular and cellular levels. For instance, it has been shown to enhance skin microvascular blood flow at the distal calf, reduce sensory symptoms, and improve measures of quality of life in patients with diabetic peripheral neuropathy .
Action Environment
The action of ruboxistaurin can be influenced by various environmental factors. For example, co-administration with known CYP3A4 inducing agents (such as rifampicin, carbamazepine, phenobarbital, etc.) may decrease the concentrations of ruboxistaurin . Therefore, the patient’s medication regimen and the timing of drug administration can significantly impact the efficacy and stability of ruboxistaurin .
Orientations Futures
Ruboxistaurin has been suggested as a potential therapeutic strategy for COVID-19 due to its ability to reduce proinflammatory and tissue-damaging consequences of neutrophils during disease . Additionally, research is being conducted to develop Ruboxistaurin nanoparticles for the treatment of diabetic retinopathy .
Propriétés
IUPAC Name |
(18S)-18-[(dimethylamino)methyl]-17-oxa-4,14,21-triazahexacyclo[19.6.1.17,14.02,6.08,13.022,27]nonacosa-1(28),2(6),7(29),8,10,12,22,24,26-nonaene-3,5-dione | |
---|---|---|
Source | PubChem | |
URL | https://pubchem.ncbi.nlm.nih.gov | |
Description | Data deposited in or computed by PubChem | |
InChI |
InChI=1S/C28H28N4O3/c1-30(2)15-18-11-12-31-16-21(19-7-3-5-9-23(19)31)25-26(28(34)29-27(25)33)22-17-32(13-14-35-18)24-10-6-4-8-20(22)24/h3-10,16-18H,11-15H2,1-2H3,(H,29,33,34)/t18-/m0/s1 | |
Source | PubChem | |
URL | https://pubchem.ncbi.nlm.nih.gov | |
Description | Data deposited in or computed by PubChem | |
InChI Key |
ZCBUQCWBWNUWSU-SFHVURJKSA-N | |
Source | PubChem | |
URL | https://pubchem.ncbi.nlm.nih.gov | |
Description | Data deposited in or computed by PubChem | |
Canonical SMILES |
CN(C)CC1CCN2C=C(C3=CC=CC=C32)C4=C(C5=CN(CCO1)C6=CC=CC=C65)C(=O)NC4=O | |
Source | PubChem | |
URL | https://pubchem.ncbi.nlm.nih.gov | |
Description | Data deposited in or computed by PubChem | |
Isomeric SMILES |
CN(C)C[C@@H]1CCN2C=C(C3=CC=CC=C32)C4=C(C5=CN(CCO1)C6=CC=CC=C65)C(=O)NC4=O | |
Source | PubChem | |
URL | https://pubchem.ncbi.nlm.nih.gov | |
Description | Data deposited in or computed by PubChem | |
Molecular Formula |
C28H28N4O3 | |
Source | PubChem | |
URL | https://pubchem.ncbi.nlm.nih.gov | |
Description | Data deposited in or computed by PubChem | |
DSSTOX Substance ID |
DTXSID00168775 | |
Record name | Ruboxistaurin | |
Source | EPA DSSTox | |
URL | https://comptox.epa.gov/dashboard/DTXSID00168775 | |
Description | DSSTox provides a high quality public chemistry resource for supporting improved predictive toxicology. | |
Molecular Weight |
468.5 g/mol | |
Source | PubChem | |
URL | https://pubchem.ncbi.nlm.nih.gov | |
Description | Data deposited in or computed by PubChem | |
CAS RN |
169939-94-0 | |
Record name | Ruboxistaurin | |
Source | CAS Common Chemistry | |
URL | https://commonchemistry.cas.org/detail?cas_rn=169939-94-0 | |
Description | CAS Common Chemistry is an open community resource for accessing chemical information. Nearly 500,000 chemical substances from CAS REGISTRY cover areas of community interest, including common and frequently regulated chemicals, and those relevant to high school and undergraduate chemistry classes. This chemical information, curated by our expert scientists, is provided in alignment with our mission as a division of the American Chemical Society. | |
Explanation | The data from CAS Common Chemistry is provided under a CC-BY-NC 4.0 license, unless otherwise stated. | |
Record name | Ruboxistaurin [INN] | |
Source | ChemIDplus | |
URL | https://pubchem.ncbi.nlm.nih.gov/substance/?source=chemidplus&sourceid=0169939940 | |
Description | ChemIDplus is a free, web search system that provides access to the structure and nomenclature authority files used for the identification of chemical substances cited in National Library of Medicine (NLM) databases, including the TOXNET system. | |
Record name | Ruboxistaurin | |
Source | DrugBank | |
URL | https://www.drugbank.ca/drugs/DB11829 | |
Description | The DrugBank database is a unique bioinformatics and cheminformatics resource that combines detailed drug (i.e. chemical, pharmacological and pharmaceutical) data with comprehensive drug target (i.e. sequence, structure, and pathway) information. | |
Explanation | Creative Common's Attribution-NonCommercial 4.0 International License (http://creativecommons.org/licenses/by-nc/4.0/legalcode) | |
Record name | Ruboxistaurin | |
Source | EPA DSSTox | |
URL | https://comptox.epa.gov/dashboard/DTXSID00168775 | |
Description | DSSTox provides a high quality public chemistry resource for supporting improved predictive toxicology. | |
Record name | RUBOXISTAURIN | |
Source | FDA Global Substance Registration System (GSRS) | |
URL | https://gsrs.ncats.nih.gov/ginas/app/beta/substances/721809WQCP | |
Description | The FDA Global Substance Registration System (GSRS) enables the efficient and accurate exchange of information on what substances are in regulated products. Instead of relying on names, which vary across regulatory domains, countries, and regions, the GSRS knowledge base makes it possible for substances to be defined by standardized, scientific descriptions. | |
Explanation | Unless otherwise noted, the contents of the FDA website (www.fda.gov), both text and graphics, are not copyrighted. They are in the public domain and may be republished, reprinted and otherwise used freely by anyone without the need to obtain permission from FDA. Credit to the U.S. Food and Drug Administration as the source is appreciated but not required. | |
Retrosynthesis Analysis
AI-Powered Synthesis Planning: Our tool employs the Template_relevance Pistachio, Template_relevance Bkms_metabolic, Template_relevance Pistachio_ringbreaker, Template_relevance Reaxys, Template_relevance Reaxys_biocatalysis model, leveraging a vast database of chemical reactions to predict feasible synthetic routes.
One-Step Synthesis Focus: Specifically designed for one-step synthesis, it provides concise and direct routes for your target compounds, streamlining the synthesis process.
Accurate Predictions: Utilizing the extensive PISTACHIO, BKMS_METABOLIC, PISTACHIO_RINGBREAKER, REAXYS, REAXYS_BIOCATALYSIS database, our tool offers high-accuracy predictions, reflecting the latest in chemical research and data.
Strategy Settings
Precursor scoring | Relevance Heuristic |
---|---|
Min. plausibility | 0.01 |
Model | Template_relevance |
Template Set | Pistachio/Bkms_metabolic/Pistachio_ringbreaker/Reaxys/Reaxys_biocatalysis |
Top-N result to add to graph | 6 |
Feasible Synthetic Routes
Avertissement et informations sur les produits de recherche in vitro
Veuillez noter que tous les articles et informations sur les produits présentés sur BenchChem sont destinés uniquement à des fins informatives. Les produits disponibles à l'achat sur BenchChem sont spécifiquement conçus pour des études in vitro, qui sont réalisées en dehors des organismes vivants. Les études in vitro, dérivées du terme latin "in verre", impliquent des expériences réalisées dans des environnements de laboratoire contrôlés à l'aide de cellules ou de tissus. Il est important de noter que ces produits ne sont pas classés comme médicaments et n'ont pas reçu l'approbation de la FDA pour la prévention, le traitement ou la guérison de toute condition médicale, affection ou maladie. Nous devons souligner que toute forme d'introduction corporelle de ces produits chez les humains ou les animaux est strictement interdite par la loi. Il est essentiel de respecter ces directives pour assurer la conformité aux normes légales et éthiques en matière de recherche et d'expérimentation.