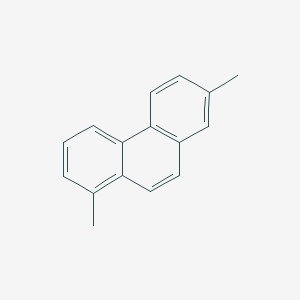
1,7-Diméthylphénanthrène
Vue d'ensemble
Description
1,7-Dimethylphenanthrene is an organic compound with the molecular formula C₁₆H₁₄. It belongs to the class of polycyclic aromatic hydrocarbons, which are composed of multiple aromatic rings. This compound is a derivative of phenanthrene, where two methyl groups are attached at the 1 and 7 positions of the phenanthrene structure .
Applications De Recherche Scientifique
1,7-Dimethylphenanthrene has several scientific research applications:
Chemistry: It is used as a model compound to study the behavior of polycyclic aromatic hydrocarbons in various chemical reactions.
Medicine: Studies on its potential biological activity and toxicity contribute to the development of new therapeutic agents.
Industry: It is used in the synthesis of advanced materials and as a precursor for other chemical compounds.
Méthodes De Préparation
Synthetic Routes and Reaction Conditions
1,7-Dimethylphenanthrene can be synthesized through various methods. One common approach involves the directed ortho metalation (DoM) of N,N-diethylbenzamides followed by Suzuki–Miyaura cross-coupling reactions . The general procedure includes:
Aryl Iodide and ortho-Bromobenzoyl Chlorides: These are reacted in the presence of norbornadiene, palladium acetate (Pd(OAc)₂), triphenylphosphine, and cesium carbonate (Cs₂CO₃) in dimethylformamide (DMF).
Reaction Conditions: The reaction is typically carried out under an inert atmosphere at elevated temperatures to facilitate the coupling process.
Industrial Production Methods
Industrial production methods for 1,7-Dimethylphenanthrene are not well-documented, likely due to its specialized applications and limited demand. the principles of large-scale organic synthesis, such as optimizing reaction conditions and using continuous flow reactors, can be applied to scale up the laboratory synthesis methods.
Analyse Des Réactions Chimiques
Types of Reactions
1,7-Dimethylphenanthrene undergoes various chemical reactions, including:
Oxidation: This compound can be oxidized to form quinones and other oxygenated derivatives.
Reduction: Reduction reactions can convert it into dihydro or tetrahydro derivatives.
Substitution: Electrophilic substitution reactions can introduce additional functional groups into the aromatic rings.
Common Reagents and Conditions
Oxidation: Common oxidizing agents include potassium permanganate (KMnO₄) and chromium trioxide (CrO₃).
Reduction: Reducing agents such as lithium aluminum hydride (LiAlH₄) and sodium borohydride (NaBH₄) are used.
Substitution: Reagents like bromine (Br₂) and nitric acid (HNO₃) are employed for halogenation and nitration reactions, respectively.
Major Products Formed
The major products formed from these reactions include:
Oxidation: Quinones and other oxygenated derivatives.
Reduction: Dihydro and tetrahydro derivatives.
Substitution: Halogenated and nitrated derivatives.
Mécanisme D'action
The mechanism of action of 1,7-Dimethylphenanthrene involves its interaction with various molecular targets and pathways:
Molecular Targets: It can interact with enzymes and receptors involved in metabolic processes.
Comparaison Avec Des Composés Similaires
1,7-Dimethylphenanthrene can be compared with other dimethylated phenanthrenes:
2,3-Dimethylphenanthrene: Similar in structure but with methyl groups at the 2 and 3 positions.
2,7-Dimethylphenanthrene: Methyl groups at the 2 and 7 positions.
2,6-Dimethylphenanthrene: Methyl groups at the 2 and 6 positions.
Uniqueness
1,7-Dimethylphenanthrene is unique due to its specific substitution pattern, which influences its chemical reactivity and physical properties. This uniqueness makes it valuable for studying the effects of methyl substitution on the behavior of polycyclic aromatic hydrocarbons .
Activité Biologique
1,7-Dimethylphenanthrene (1,7-DMP) is a polycyclic aromatic hydrocarbon (PAH) with the molecular formula C₁₆H₁₄. It consists of three fused benzene rings with methyl groups at the 1 and 7 positions. This compound is of significant interest due to its potential biological activities, including toxicity, mutagenicity, and effects on various biological systems.
Chemical Structure
The structure of 1,7-Dimethylphenanthrene can be represented as follows:
Synthesis Methods
1,7-Dimethylphenanthrene can be synthesized through several methods, including:
- Directed Ortho Metalation (DoM) : Involves the metalation of N,N-diethylbenzamides followed by Suzuki–Miyaura cross-coupling reactions.
- Industrial Production : While not extensively documented, principles of large-scale organic synthesis can be applied to optimize reaction conditions for this compound.
Toxicity and Environmental Impact
Research indicates that 1,7-DMP exhibits varying degrees of toxicity. For instance, studies have shown that it can affect aquatic life stages, particularly in fish embryos. A study measuring the toxicity of alkyl-phenanthrenes found that 1,7-DMP was a weak inducer of cytochrome P450 1A (CYP1A) and was capable of binding to the aryl hydrocarbon receptor (AHR), suggesting a mechanism for its toxic effects .
Table 1: Toxicity Data for 1,7-Dimethylphenanthrene
Study | Organism | Effect | Concentration | Reference |
---|---|---|---|---|
A | Medaka fish embryos | Toxicity observed | Varies | |
B | Aquatic organisms | Weak CYP1A induction | N/A | |
C | Fish embryos | No significant toxicity at low concentrations | N/A |
Metabolic Activation
The biological activity of 1,7-DMP is largely attributed to its metabolic activation, which leads to the formation of reactive intermediates. These intermediates can interact with cellular macromolecules such as DNA and proteins, potentially causing mutagenic effects .
Biodegradation Studies
Biodegradation studies indicate that demethylation is a critical process in the breakdown of alkylphenanthrenes like 1,7-DMP in contaminated environments. The compound's resistance to biodegradation varies significantly based on environmental conditions and microbial communities present .
Case Study 1: Toxicity to Early Life Stages
In a study assessing the toxicity of various alkyl-phenanthrenes to early life stages of medaka fish, 1,7-DMP was included among several test compounds. The results indicated that while it exhibited some level of toxicity, it was less harmful compared to other PAHs with different alkyl substitutions .
Case Study 2: Environmental Monitoring
Monitoring studies in lakes affected by petroleum combustion revealed varying concentrations of PAHs including 1,7-DMP. The findings suggested that while some lakes showed significant contamination levels leading to ecological impacts, others remained relatively unaffected due to lower deposition rates .
The mechanism through which 1,7-Dimethylphenanthrene exerts its biological effects involves:
- Interaction with AHR : Binding to AHR leads to the activation of gene expression related to xenobiotic metabolism.
- Induction of CYP Enzymes : Induction of CYP enzymes may result in increased metabolic activation and subsequent toxicity.
Propriétés
IUPAC Name |
1,7-dimethylphenanthrene | |
---|---|---|
Source | PubChem | |
URL | https://pubchem.ncbi.nlm.nih.gov | |
Description | Data deposited in or computed by PubChem | |
InChI |
InChI=1S/C16H14/c1-11-6-8-15-13(10-11)7-9-14-12(2)4-3-5-16(14)15/h3-10H,1-2H3 | |
Source | PubChem | |
URL | https://pubchem.ncbi.nlm.nih.gov | |
Description | Data deposited in or computed by PubChem | |
InChI Key |
NZCMUISOTIPJAM-UHFFFAOYSA-N | |
Source | PubChem | |
URL | https://pubchem.ncbi.nlm.nih.gov | |
Description | Data deposited in or computed by PubChem | |
Canonical SMILES |
CC1=CC2=C(C=C1)C3=CC=CC(=C3C=C2)C | |
Source | PubChem | |
URL | https://pubchem.ncbi.nlm.nih.gov | |
Description | Data deposited in or computed by PubChem | |
Molecular Formula |
C16H14 | |
Source | PubChem | |
URL | https://pubchem.ncbi.nlm.nih.gov | |
Description | Data deposited in or computed by PubChem | |
DSSTOX Substance ID |
DTXSID1074755 | |
Record name | Phenanthrene, 1,7-dimethyl- | |
Source | EPA DSSTox | |
URL | https://comptox.epa.gov/dashboard/DTXSID1074755 | |
Description | DSSTox provides a high quality public chemistry resource for supporting improved predictive toxicology. | |
Molecular Weight |
206.28 g/mol | |
Source | PubChem | |
URL | https://pubchem.ncbi.nlm.nih.gov | |
Description | Data deposited in or computed by PubChem | |
CAS No. |
483-87-4 | |
Record name | Phenanthrene, 1,7-dimethyl- | |
Source | ChemIDplus | |
URL | https://pubchem.ncbi.nlm.nih.gov/substance/?source=chemidplus&sourceid=0000483874 | |
Description | ChemIDplus is a free, web search system that provides access to the structure and nomenclature authority files used for the identification of chemical substances cited in National Library of Medicine (NLM) databases, including the TOXNET system. | |
Record name | Phenanthrene, 1,7-dimethyl- | |
Source | EPA DSSTox | |
URL | https://comptox.epa.gov/dashboard/DTXSID1074755 | |
Description | DSSTox provides a high quality public chemistry resource for supporting improved predictive toxicology. | |
Record name | Pimanthrene | |
Source | FDA Global Substance Registration System (GSRS) | |
URL | https://gsrs.ncats.nih.gov/ginas/app/beta/substances/665XXE9T4V | |
Description | The FDA Global Substance Registration System (GSRS) enables the efficient and accurate exchange of information on what substances are in regulated products. Instead of relying on names, which vary across regulatory domains, countries, and regions, the GSRS knowledge base makes it possible for substances to be defined by standardized, scientific descriptions. | |
Explanation | Unless otherwise noted, the contents of the FDA website (www.fda.gov), both text and graphics, are not copyrighted. They are in the public domain and may be republished, reprinted and otherwise used freely by anyone without the need to obtain permission from FDA. Credit to the U.S. Food and Drug Administration as the source is appreciated but not required. | |
Retrosynthesis Analysis
AI-Powered Synthesis Planning: Our tool employs the Template_relevance Pistachio, Template_relevance Bkms_metabolic, Template_relevance Pistachio_ringbreaker, Template_relevance Reaxys, Template_relevance Reaxys_biocatalysis model, leveraging a vast database of chemical reactions to predict feasible synthetic routes.
One-Step Synthesis Focus: Specifically designed for one-step synthesis, it provides concise and direct routes for your target compounds, streamlining the synthesis process.
Accurate Predictions: Utilizing the extensive PISTACHIO, BKMS_METABOLIC, PISTACHIO_RINGBREAKER, REAXYS, REAXYS_BIOCATALYSIS database, our tool offers high-accuracy predictions, reflecting the latest in chemical research and data.
Strategy Settings
Precursor scoring | Relevance Heuristic |
---|---|
Min. plausibility | 0.01 |
Model | Template_relevance |
Template Set | Pistachio/Bkms_metabolic/Pistachio_ringbreaker/Reaxys/Reaxys_biocatalysis |
Top-N result to add to graph | 6 |
Feasible Synthetic Routes
Q1: What are the primary sources of 1,7-Dimethylphenanthrene in the environment?
A1: 1,7-Dimethylphenanthrene is primarily generated through the combustion of organic matter, particularly wood. Research indicates a strong association between 1,7-Dimethylphenanthrene and the burning of softwoods like pines. [] This compound is often found in higher concentrations in areas with significant wood combustion activity. [] Additionally, 1,7-Dimethylphenanthrene has been identified in coal and coaly shale deposits, suggesting its origin from ancient plant matter, specifically coniferous gymnosperms. []
Q2: How does the presence of 1,7-Dimethylphenanthrene in sediment cores help us understand historical pollution trends?
A2: 1,7-Dimethylphenanthrene serves as a valuable marker for tracing historical pollution trends, particularly those related to wood combustion. [] Analyzing its presence in sediment cores allows researchers to reconstruct past combustion activities. For example, a study of sediment cores from Central Park Lake in New York City revealed a peak in 1,7-Dimethylphenanthrene levels corresponding to periods of increased coal usage in the city's history. [] This information aids in understanding the long-term impact of human activities on the environment.
Q3: Can 1,7-Dimethylphenanthrene help differentiate between various combustion sources?
A3: Yes, the ratio of 1,7-Dimethylphenanthrene to other Dimethylphenanthrene isomers can be used to differentiate between combustion sources. For instance, a higher ratio of 1,7-Dimethylphenanthrene to 2,6-Dimethylphenanthrene suggests a greater contribution from residential wood combustion, especially of softwoods. [] This ratio proves useful in source apportionment studies, helping determine the relative contributions of various sources to overall pollution levels.
Q4: Are there any specific analytical techniques used to quantify 1,7-Dimethylphenanthrene in environmental samples?
A4: Gas chromatography coupled with mass spectrometry (GC-MS) is a widely employed technique for quantifying 1,7-Dimethylphenanthrene in environmental matrices like air particulate matter and sediment samples. [, ] This method provides high sensitivity and selectivity, enabling the separation and detection of 1,7-Dimethylphenanthrene from other compounds in complex mixtures.
Q5: What is the significance of 1,7-Dimethylphenanthrene in studying Black Carbon sequestration?
A5: 1,7-Dimethylphenanthrene, alongside other combustion markers, aids in understanding Black Carbon sequestration in sediments. [] Its presence, especially in conjunction with the 1,7-Dimethylphenanthrene to 2,6-Dimethylphenanthrene ratio, can help distinguish between fossil fuel and wood combustion as sources of Black Carbon. This distinction is crucial for assessing the impact of Black Carbon on the global carbon cycle. For instance, Black Carbon from wood combustion, unlike that from fossil fuels, represents a transfer of carbon from the biosphere to the geosphere. []
Q6: Is 1,7-Dimethylphenanthrene used as a biomarker in any other research areas?
A6: Beyond pollution studies, 1,7-Dimethylphenanthrene finds application as a biomarker in organic geochemistry. Its presence in geological formations, especially alongside other organic compounds like retene, can provide insights into the depositional environment and source of organic matter in ancient sediments. [, , ] This information is valuable for understanding past climates and ecosystems.
Q7: Are there any known challenges or limitations associated with using 1,7-Dimethylphenanthrene as a marker in research?
A7: One challenge in using 1,7-Dimethylphenanthrene as a marker lies in the potential for its degradation in the environment. While relatively stable, it can undergo degradation processes like biodegradation, which may complicate the interpretation of its presence and abundance in certain contexts. [] Additionally, the influence of factors like organic matter source and maturity level on 1,7-Dimethylphenanthrene levels should be carefully considered during data interpretation. [, ]
Avertissement et informations sur les produits de recherche in vitro
Veuillez noter que tous les articles et informations sur les produits présentés sur BenchChem sont destinés uniquement à des fins informatives. Les produits disponibles à l'achat sur BenchChem sont spécifiquement conçus pour des études in vitro, qui sont réalisées en dehors des organismes vivants. Les études in vitro, dérivées du terme latin "in verre", impliquent des expériences réalisées dans des environnements de laboratoire contrôlés à l'aide de cellules ou de tissus. Il est important de noter que ces produits ne sont pas classés comme médicaments et n'ont pas reçu l'approbation de la FDA pour la prévention, le traitement ou la guérison de toute condition médicale, affection ou maladie. Nous devons souligner que toute forme d'introduction corporelle de ces produits chez les humains ou les animaux est strictement interdite par la loi. Il est essentiel de respecter ces directives pour assurer la conformité aux normes légales et éthiques en matière de recherche et d'expérimentation.