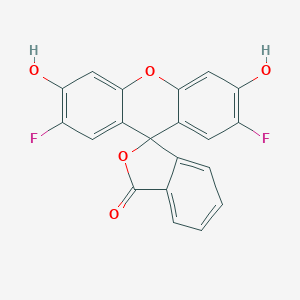
2',7'-Difluorofluorescéine
Vue d'ensemble
Description
2’,7’-Difluorofluorescein is a xanthene dye that is structurally similar to fluorescein but with two fluoro substituents at positions 2’ and 7’. This compound is known for its fluorescent properties and is commonly used as a fluorescent probe in various scientific applications .
Applications De Recherche Scientifique
2’,7’-Difluorofluorescein has a wide range of applications in scientific research:
Chemistry: Used as a fluorescent probe to study chemical reactions and molecular interactions.
Biology: Employed in fluorescence microscopy to stain biological specimens and visualize cellular structures.
Medicine: Utilized in diagnostic assays to detect specific biomolecules and monitor physiological processes.
Industry: Applied in the development of fluorescent dyes and sensors for various industrial applications .
Analyse Biochimique
Biochemical Properties
2’,7’-Difluorofluorescein plays a crucial role in biochemical reactions as a fluorescent probe. It interacts with reactive oxygen species, such as hydrogen peroxide, superoxide, and hydroxyl radicals. Upon interaction with these species, 2’,7’-Difluorofluorescein undergoes oxidation, resulting in a fluorescent product that can be detected and quantified using fluorescence microscopy or spectroscopy. This property makes it an essential tool for studying oxidative stress and related cellular processes. Additionally, 2’,7’-Difluorofluorescein can interact with various enzymes, including peroxidases, which catalyze its oxidation, further enhancing its utility in biochemical assays .
Cellular Effects
2’,7’-Difluorofluorescein has significant effects on various types of cells and cellular processes. It is widely used to monitor oxidative stress in cells, as its fluorescence increases in the presence of reactive oxygen species. This compound influences cell function by providing insights into the levels of oxidative stress, which can affect cell signaling pathways, gene expression, and cellular metabolism. For instance, increased oxidative stress detected by 2’,7’-Difluorofluorescein can lead to the activation of stress response pathways, alterations in gene expression, and changes in metabolic activity .
Molecular Mechanism
The molecular mechanism of 2’,7’-Difluorofluorescein involves its oxidation by reactive oxygen species. Once inside the cell, 2’,7’-Difluorofluorescein is deacetylated by cytosolic esterases to form the non-fluorescent 2’,7’-difluorodihydrofluorescein. This intermediate is then oxidized by reactive oxygen species to produce the fluorescent 2’,7’-difluorofluorescein. This oxidation process is crucial for its function as a probe, as the fluorescence intensity correlates with the levels of reactive oxygen species, allowing for real-time monitoring of oxidative stress within cells .
Temporal Effects in Laboratory Settings
In laboratory settings, the effects of 2’,7’-Difluorofluorescein can change over time. The stability of this compound is influenced by factors such as light exposure and temperature. It is generally stable when stored in the dark at low temperatures, but prolonged exposure to light can lead to degradation and reduced fluorescence. In in vitro studies, the fluorescence signal of 2’,7’-Difluorofluorescein can be used to monitor oxidative stress over time, providing valuable information on the dynamics of cellular responses to oxidative stress .
Dosage Effects in Animal Models
The effects of 2’,7’-Difluorofluorescein vary with different dosages in animal models. At low doses, it is effective in detecting oxidative stress without causing significant toxicity. At higher doses, it may exhibit toxic effects, including cellular damage and alterations in normal cellular functions. It is essential to optimize the dosage to achieve accurate detection of oxidative stress while minimizing adverse effects. Studies have shown that the threshold for toxicity varies depending on the animal model and the specific experimental conditions .
Metabolic Pathways
2’,7’-Difluorofluorescein is involved in metabolic pathways related to oxidative stress. It interacts with enzymes such as peroxidases, which catalyze its oxidation. This interaction is crucial for its function as a fluorescent probe, as it allows for the detection of reactive oxygen species. The metabolic pathways involving 2’,7’-Difluorofluorescein are essential for understanding the cellular responses to oxidative stress and the role of reactive oxygen species in various physiological and pathological processes .
Transport and Distribution
Within cells, 2’,7’-Difluorofluorescein is transported and distributed through passive diffusion across cellular membranes. Once inside the cell, it is deacetylated by cytosolic esterases to form the non-fluorescent intermediate, which is then oxidized to the fluorescent form. The distribution of 2’,7’-Difluorofluorescein within cells is influenced by factors such as membrane permeability and the presence of reactive oxygen species. Its localization and accumulation within specific cellular compartments can provide insights into the spatial dynamics of oxidative stress .
Subcellular Localization
The subcellular localization of 2’,7’-Difluorofluorescein is primarily in the cytoplasm, where it interacts with reactive oxygen species. It can also accumulate in other cellular compartments, such as the mitochondria and the nucleus, depending on the levels of oxidative stress and the specific experimental conditions. The localization of 2’,7’-Difluorofluorescein is crucial for its function as a probe, as it allows for the detection of reactive oxygen species in different cellular compartments, providing a comprehensive view of oxidative stress within cells .
Méthodes De Préparation
Synthetic Routes and Reaction Conditions
The synthesis of 2’,7’-Difluorofluorescein typically involves the fluorination of fluorescein. One common method is the reaction of fluorescein with a fluorinating agent such as diethylaminosulfur trifluoride (DAST) under controlled conditions. The reaction is usually carried out in an inert atmosphere to prevent unwanted side reactions .
Industrial Production Methods
Industrial production of 2’,7’-Difluorofluorescein follows similar synthetic routes but on a larger scale. The process involves the use of high-purity reagents and stringent quality control measures to ensure the consistency and purity of the final product. The compound is then purified using techniques such as recrystallization or chromatography .
Analyse Des Réactions Chimiques
Types of Reactions
2’,7’-Difluorofluorescein undergoes various chemical reactions, including:
Oxidation: The compound can be oxidized to form different fluorescent derivatives.
Reduction: Reduction reactions can modify the fluorescence properties of the compound.
Substitution: The fluoro groups can be substituted with other functional groups to create new derivatives.
Common Reagents and Conditions
Oxidation: Common oxidizing agents include hydrogen peroxide and potassium permanganate.
Reduction: Reducing agents such as sodium borohydride are often used.
Substitution: Reagents like sodium hydride and alkyl halides are used for substitution reactions.
Major Products Formed
The major products formed from these reactions include various fluorescent derivatives that have different emission spectra and intensities. These derivatives are useful in different scientific applications .
Mécanisme D'action
The mechanism of action of 2’,7’-Difluorofluorescein involves its ability to fluoresce upon excitation by light. The compound absorbs light at a specific wavelength and emits light at a longer wavelength, which can be detected using fluorescence spectroscopy. This property makes it an excellent tool for detecting and quantifying various analytes in biological and chemical systems .
Comparaison Avec Des Composés Similaires
Similar Compounds
2’,7’-Dichlorofluorescein: Similar in structure but with chlorine substituents instead of fluorine.
Fluorescein: The parent compound without any halogen substituents.
2’,7’-Dibromofluorescein: Contains bromine substituents instead of fluorine
Uniqueness
2’,7’-Difluorofluorescein is unique due to its specific fluorescent properties, which are influenced by the presence of fluorine atoms. These properties make it particularly useful in applications where high sensitivity and specificity are required .
Activité Biologique
2',7'-Difluorofluorescein (DF) is a fluorescent compound widely used as a probe for detecting reactive oxygen species (ROS) in biological systems. This article provides a comprehensive overview of its biological activity, including its mechanisms of action, applications in research, and relevant case studies.
Overview of 2',7'-Difluorofluorescein
2',7'-Difluorofluorescein is a derivative of fluorescein, characterized by the substitution of two fluorine atoms at the 2' and 7' positions. This modification enhances its fluorescent properties and stability in biological environments, making it suitable for various applications in cellular biology and biochemistry.
The primary mechanism by which DF operates involves the oxidation of the non-fluorescent precursor, 2',7'-dichlorodihydrofluorescein (DCFH), to produce the highly fluorescent compound DF. This reaction occurs in the presence of ROS, such as hydrogen peroxide (), superoxide anions (), and hydroxyl radicals (). The fluorescence intensity of DF is directly proportional to the concentration of ROS present, allowing for quantitative measurements of oxidative stress within cells.
Applications in Biological Research
DF is extensively utilized in various biological contexts, including:
- Oxidative Stress Measurement : DF serves as a reliable indicator for assessing oxidative stress levels in different cell types and tissues.
- Cell Viability Assays : It is employed to evaluate the impact of oxidative stress on cell viability and function.
- Pathophysiological Studies : DF has been used in studies investigating conditions such as ischemia-reperfusion injury, neurodegenerative diseases, and cancer.
Case Study 1: Detection of ROS in Hepatocytes
A study utilized DCFH to measure ROS production in hepatocytes subjected to mitochondrial stress induced by rotenone. The results indicated that DCF fluorescence intensity correlated with increased oxidative stress levels, demonstrating the utility of DF as a probe for mitochondrial dysfunction .
Case Study 2: UVA-Induced Damage
In another investigation, cells loaded with DCFH were exposed to UVA radiation to study ROS generation. The appearance of DF fluorescence was monitored, confirming that UVA exposure resulted in significant ROS production, further establishing DCFH's role in assessing oxidative damage .
Case Study 3: Liposomal Delivery Systems
Recent advancements have explored liposomal formulations of DCFH for targeted delivery during photodynamic therapy. This approach enabled real-time visualization of oxidant production during liver ischemia-reperfusion, highlighting the versatility of DF in therapeutic applications .
Comparative Analysis of Fluorescent Probes
The following table summarizes key characteristics of various fluorescent probes used for ROS detection:
Probe Name | Mechanism | Fluorescence Emission (nm) | Specificity | Applications |
---|---|---|---|---|
2',7'-Difluorofluorescein | Oxidation by ROS | 523 | General ROS detection | Cell viability, oxidative stress |
Dihydroethidium | Reaction with superoxide | Variable | Superoxide specific | Cellular redox state assessment |
Amplex Red | Reaction with hydrogen peroxide | 582 | H2O2 specific | Enzyme assays, cellular signaling |
Propriétés
IUPAC Name |
2',7'-difluoro-3',6'-dihydroxyspiro[2-benzofuran-3,9'-xanthene]-1-one | |
---|---|---|
Source | PubChem | |
URL | https://pubchem.ncbi.nlm.nih.gov | |
Description | Data deposited in or computed by PubChem | |
InChI |
InChI=1S/C20H10F2O5/c21-13-5-11-17(7-15(13)23)26-18-8-16(24)14(22)6-12(18)20(11)10-4-2-1-3-9(10)19(25)27-20/h1-8,23-24H | |
Source | PubChem | |
URL | https://pubchem.ncbi.nlm.nih.gov | |
Description | Data deposited in or computed by PubChem | |
InChI Key |
FJXJIUHGLVUXQP-UHFFFAOYSA-N | |
Source | PubChem | |
URL | https://pubchem.ncbi.nlm.nih.gov | |
Description | Data deposited in or computed by PubChem | |
Canonical SMILES |
C1=CC=C2C(=C1)C(=O)OC23C4=CC(=C(C=C4OC5=CC(=C(C=C35)F)O)O)F | |
Source | PubChem | |
URL | https://pubchem.ncbi.nlm.nih.gov | |
Description | Data deposited in or computed by PubChem | |
Molecular Formula |
C20H10F2O5 | |
Source | PubChem | |
URL | https://pubchem.ncbi.nlm.nih.gov | |
Description | Data deposited in or computed by PubChem | |
Molecular Weight |
368.3 g/mol | |
Source | PubChem | |
URL | https://pubchem.ncbi.nlm.nih.gov | |
Description | Data deposited in or computed by PubChem | |
Retrosynthesis Analysis
AI-Powered Synthesis Planning: Our tool employs the Template_relevance Pistachio, Template_relevance Bkms_metabolic, Template_relevance Pistachio_ringbreaker, Template_relevance Reaxys, Template_relevance Reaxys_biocatalysis model, leveraging a vast database of chemical reactions to predict feasible synthetic routes.
One-Step Synthesis Focus: Specifically designed for one-step synthesis, it provides concise and direct routes for your target compounds, streamlining the synthesis process.
Accurate Predictions: Utilizing the extensive PISTACHIO, BKMS_METABOLIC, PISTACHIO_RINGBREAKER, REAXYS, REAXYS_BIOCATALYSIS database, our tool offers high-accuracy predictions, reflecting the latest in chemical research and data.
Strategy Settings
Precursor scoring | Relevance Heuristic |
---|---|
Min. plausibility | 0.01 |
Model | Template_relevance |
Template Set | Pistachio/Bkms_metabolic/Pistachio_ringbreaker/Reaxys/Reaxys_biocatalysis |
Top-N result to add to graph | 6 |
Feasible Synthetic Routes
Avertissement et informations sur les produits de recherche in vitro
Veuillez noter que tous les articles et informations sur les produits présentés sur BenchChem sont destinés uniquement à des fins informatives. Les produits disponibles à l'achat sur BenchChem sont spécifiquement conçus pour des études in vitro, qui sont réalisées en dehors des organismes vivants. Les études in vitro, dérivées du terme latin "in verre", impliquent des expériences réalisées dans des environnements de laboratoire contrôlés à l'aide de cellules ou de tissus. Il est important de noter que ces produits ne sont pas classés comme médicaments et n'ont pas reçu l'approbation de la FDA pour la prévention, le traitement ou la guérison de toute condition médicale, affection ou maladie. Nous devons souligner que toute forme d'introduction corporelle de ces produits chez les humains ou les animaux est strictement interdite par la loi. Il est essentiel de respecter ces directives pour assurer la conformité aux normes légales et éthiques en matière de recherche et d'expérimentation.