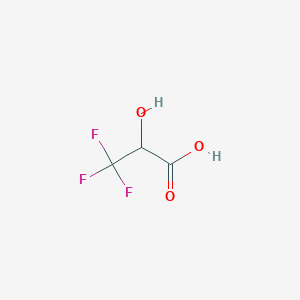
3,3,3-Trifluoro-2-hydroxypropanoic acid
Vue d'ensemble
Description
3,3,3-Trifluoro-2-hydroxypropanoic acid, also known as TFHCA, is a fluorinated organic compound. It has the molecular formula C3H3F3O3 and a molecular weight of 144.05 g/mol . The compound has gained attention in recent years due to its unique physical, chemical, and biological properties.
Synthesis Analysis
The synthesis of similar compounds has been reported in the literature. For instance, the synthesis of 3,3,3-Trifluoro-2,2-dimethylpropionic Acid was achieved through fluoridation, hydrolysis, and acidification reactions, with an overall yield of 49.6% . The influence of time, temperature, amount of SF4 on the fluoridation, and amount of CH2Cl2, the time on the hydrolysis reaction were investigated .
Molecular Structure Analysis
The molecular structure of 3,3,3-Trifluoro-2-hydroxypropanoic acid can be represented by the SMILES string OC(C(O)=O)C(F)(F)F
. The InChI representation is InChI=1S/C3H3F3O3/c4-3(5,6)1(7)2(8)9/h1,7H,(H,8,9)
.
Applications De Recherche Scientifique
Biocatalysis
TFHPA: serves as a substrate for biocatalytic processes. A study identified a thermostable and cobalt-dependent amidase from Burkholderia phytofirmans that efficiently synthesizes ®-3,3,3-trifluoro-2-hydroxy-2-methylpropionic acid , an important chiral building block for pharmaceuticals . This enzyme showcases high substrate tolerance and activity, making it a valuable tool for industrial biocatalysis.
Pharmaceutical Synthesis
The compound is a precursor for synthesizing enantiomerically pure acids, crucial for creating pharmaceuticals with specific desired effects. The stereochemistry of drug molecules can significantly influence their efficacy and safety .
Polymer Production
TFHPA: is a potential monomer for producing fluorinated polymers. These polymers could have unique properties, such as increased resistance to solvents and chemicals, making them suitable for specialized applications in coatings and materials science .
Safety and Hazards
Safety information for 3,3,3-Trifluoro-2-hydroxypropanoic acid indicates that it may cause skin irritation (H315), serious eye irritation (H319), and may cause respiratory irritation (H335) . Precautionary measures include avoiding breathing mist, gas or vapors, avoiding contact with skin and eyes, and using personal protective equipment .
Propriétés
IUPAC Name |
3,3,3-trifluoro-2-hydroxypropanoic acid | |
---|---|---|
Source | PubChem | |
URL | https://pubchem.ncbi.nlm.nih.gov | |
Description | Data deposited in or computed by PubChem | |
InChI |
InChI=1S/C3H3F3O3/c4-3(5,6)1(7)2(8)9/h1,7H,(H,8,9) | |
Source | PubChem | |
URL | https://pubchem.ncbi.nlm.nih.gov | |
Description | Data deposited in or computed by PubChem | |
InChI Key |
BVKGUTLIPHZYCX-UHFFFAOYSA-N | |
Source | PubChem | |
URL | https://pubchem.ncbi.nlm.nih.gov | |
Description | Data deposited in or computed by PubChem | |
Canonical SMILES |
C(C(=O)O)(C(F)(F)F)O | |
Source | PubChem | |
URL | https://pubchem.ncbi.nlm.nih.gov | |
Description | Data deposited in or computed by PubChem | |
Molecular Formula |
C3H3F3O3 | |
Source | PubChem | |
URL | https://pubchem.ncbi.nlm.nih.gov | |
Description | Data deposited in or computed by PubChem | |
Molecular Weight |
144.05 g/mol | |
Source | PubChem | |
URL | https://pubchem.ncbi.nlm.nih.gov | |
Description | Data deposited in or computed by PubChem | |
Product Name |
3,3,3-Trifluoro-2-hydroxypropanoic acid | |
CAS RN |
684-07-1 | |
Record name | 684-07-1 | |
Source | DTP/NCI | |
URL | https://dtp.cancer.gov/dtpstandard/servlet/dwindex?searchtype=NSC&outputformat=html&searchlist=56231 | |
Description | The NCI Development Therapeutics Program (DTP) provides services and resources to the academic and private-sector research communities worldwide to facilitate the discovery and development of new cancer therapeutic agents. | |
Explanation | Unless otherwise indicated, all text within NCI products is free of copyright and may be reused without our permission. Credit the National Cancer Institute as the source. | |
Record name | 3,3,3-trifluoro-2-hydroxypropanoic acid | |
Source | European Chemicals Agency (ECHA) | |
URL | https://echa.europa.eu/information-on-chemicals | |
Description | The European Chemicals Agency (ECHA) is an agency of the European Union which is the driving force among regulatory authorities in implementing the EU's groundbreaking chemicals legislation for the benefit of human health and the environment as well as for innovation and competitiveness. | |
Explanation | Use of the information, documents and data from the ECHA website is subject to the terms and conditions of this Legal Notice, and subject to other binding limitations provided for under applicable law, the information, documents and data made available on the ECHA website may be reproduced, distributed and/or used, totally or in part, for non-commercial purposes provided that ECHA is acknowledged as the source: "Source: European Chemicals Agency, http://echa.europa.eu/". Such acknowledgement must be included in each copy of the material. ECHA permits and encourages organisations and individuals to create links to the ECHA website under the following cumulative conditions: Links can only be made to webpages that provide a link to the Legal Notice page. | |
Retrosynthesis Analysis
AI-Powered Synthesis Planning: Our tool employs the Template_relevance Pistachio, Template_relevance Bkms_metabolic, Template_relevance Pistachio_ringbreaker, Template_relevance Reaxys, Template_relevance Reaxys_biocatalysis model, leveraging a vast database of chemical reactions to predict feasible synthetic routes.
One-Step Synthesis Focus: Specifically designed for one-step synthesis, it provides concise and direct routes for your target compounds, streamlining the synthesis process.
Accurate Predictions: Utilizing the extensive PISTACHIO, BKMS_METABOLIC, PISTACHIO_RINGBREAKER, REAXYS, REAXYS_BIOCATALYSIS database, our tool offers high-accuracy predictions, reflecting the latest in chemical research and data.
Strategy Settings
Precursor scoring | Relevance Heuristic |
---|---|
Min. plausibility | 0.01 |
Model | Template_relevance |
Template Set | Pistachio/Bkms_metabolic/Pistachio_ringbreaker/Reaxys/Reaxys_biocatalysis |
Top-N result to add to graph | 6 |
Feasible Synthetic Routes
Q & A
Q1: What is the molecular formula and weight of 3,3,3-Trifluoro-2-hydroxypropanoic acid?
A1: 3,3,3-Trifluoro-2-hydroxypropanoic acid, also known as rac-3,3,3-Trifluorolactic acid, has the molecular formula C3H3F3O3 []. The molecular weight can be calculated as 142.03 g/mol.
Q2: What are some key structural features of 3,3,3-Trifluoro-2-hydroxypropanoic acid revealed by its crystal structure?
A2: The crystal structure analysis of 3,3,3-Trifluoro-2-hydroxypropanoic acid reveals an O=C—C—O(H) torsion angle of 13.26 (15)° []. Furthermore, the crystal packing is stabilized by O—H⋯O hydrogen bonds and C—H⋯O contacts, which connect the molecules into sheets perpendicular to the c axis [].
Avertissement et informations sur les produits de recherche in vitro
Veuillez noter que tous les articles et informations sur les produits présentés sur BenchChem sont destinés uniquement à des fins informatives. Les produits disponibles à l'achat sur BenchChem sont spécifiquement conçus pour des études in vitro, qui sont réalisées en dehors des organismes vivants. Les études in vitro, dérivées du terme latin "in verre", impliquent des expériences réalisées dans des environnements de laboratoire contrôlés à l'aide de cellules ou de tissus. Il est important de noter que ces produits ne sont pas classés comme médicaments et n'ont pas reçu l'approbation de la FDA pour la prévention, le traitement ou la guérison de toute condition médicale, affection ou maladie. Nous devons souligner que toute forme d'introduction corporelle de ces produits chez les humains ou les animaux est strictement interdite par la loi. Il est essentiel de respecter ces directives pour assurer la conformité aux normes légales et éthiques en matière de recherche et d'expérimentation.