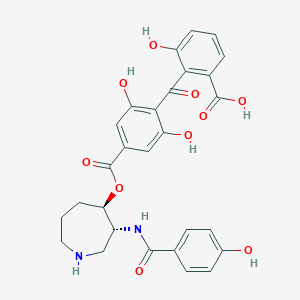
Balanol
Vue d'ensemble
Description
Balanol est un métabolite fongique produit par le champignon Verticillium balanoides. C'est un puissant inhibiteur des protéines kinases sérine/thréonine protéine kinase A (PKA) et protéine kinase C (PKC), se liant de manière similaire à l'ATP . This compound a été découvert en 1993 lors de la recherche de nouveaux inhibiteurs de la PKC, une famille de kinases sérine/thréonine dont la suractivation est associée à de nombreuses maladies humaines, notamment le cancer .
Méthodes De Préparation
Voies de synthèse et conditions réactionnelles : Balanol peut être synthétisé par une série de réactions chimiques impliquant le couplage de la benzophénone, de l'hexahydroazepane et de motifs 4-hydroxybenzoyle. Les motifs benzophénone et hexahydroazepane sont liés par une liaison ester, tandis que les motifs azepane et benzoyle sont liés par une liaison amide . La voie de synthèse implique de multiples étapes, notamment la protection et la déprotection de groupes fonctionnels, des réactions de couplage et des processus de purification.
Méthodes de production industrielle : La production industrielle de this compound implique l'optimisation des conditions de fermentation en utilisant le champignon Tolypocladium ophioglossoides. La production peut être améliorée par la surexpression du gène régulateur situé dans le cluster blnR, qui régule positivement la biosynthèse du this compound . Le milieu et les conditions de fermentation optimisés peuvent conduire à une production au niveau du gramme de this compound .
Analyse Des Réactions Chimiques
Types de réactions : Balanol subit diverses réactions chimiques, notamment des réactions d'oxydation, de réduction et de substitution. Ces réactions sont essentielles pour modifier la structure moléculaire de this compound afin d'améliorer son affinité de liaison et sa sélectivité pour des protéines kinases spécifiques.
Réactifs et conditions courants : Les réactifs courants utilisés dans les réactions chimiques du this compound comprennent les agents oxydants, les agents réducteurs et les nucléophiles pour les réactions de substitution. Les conditions réactionnelles impliquent généralement des températures contrôlées, des niveaux de pH et des systèmes de solvants pour garantir les transformations chimiques souhaitées.
Produits majeurs : Les produits majeurs formés à partir des réactions chimiques du this compound comprennent ses analogues et ses dérivés, qui sont conçus pour améliorer ses propriétés pharmacologiques et sa sélectivité pour des kinases spécifiques .
Applications de recherche scientifique
This compound a de nombreuses applications de recherche scientifique, en particulier dans les domaines de la chimie, de la biologie, de la médecine et de l'industrie. Il est utilisé comme un puissant inhibiteur ATP-compétitif de la protéine kinase C (PKC) et de la protéine kinase A (PKA), ce qui le rend précieux pour l'étude des voies de transduction du signal et des maladies liées aux kinases . This compound et ses analogues sont également utilisés pour étudier les rôles de la sélectivité et de la flexibilité des protéines dans l'inhibition des kinases .
Mécanisme d'action
This compound exerce ses effets en se liant au site de liaison de l'ATP des kinases sérine/thréonine, en particulier la protéine kinase A (PKA) et la protéine kinase C (PKC) . La liaison du this compound à ces kinases inhibe leur activité, perturbant ainsi la phosphorylation des protéines cibles impliquées dans les voies de transduction du signal . Les cibles moléculaires du this compound comprennent les sous-unités catalytiques de la PKA et de la PKC, et son mécanisme de liaison implique des interactions avec des résidus d'acides aminés clés dans le site de liaison de l'ATP .
Applications De Recherche Scientifique
Inhibition of Protein Kinase C
Balanol's primary application is in the inhibition of PKC, particularly the PKCε isozyme, which is often implicated in cancer progression. Research has demonstrated that selective inhibition of PKCε can provide a therapeutic advantage in treating various cancers.
- Case Study: Fluorinated this compound Analogues
Recent studies have focused on synthesizing fluorinated analogues of this compound to enhance selectivity towards PKCε. These analogues show improved binding affinity and selectivity due to stereospecific fluorination at specific positions on the molecule. For instance, a study highlighted that a C5(S)-fluorinated this compound analogue exhibited significantly better selectivity for PKCε compared to other PKC isozymes .
Structure-Activity Relationship Studies
Extensive structure-activity relationship (SAR) studies have been conducted to understand how modifications to the this compound structure influence its inhibitory activity and selectivity:
- Key Findings
- Charge States : The charge states of this compound and its analogues play a crucial role in their binding interactions with PKA and PKCε. Understanding these charge states through molecular dynamics (MD) simulations has been vital for rational drug design .
- Molecular Docking : Molecular docking studies have provided insights into the binding modes of this compound and its analogues within the ATP-binding sites of kinases, revealing how structural modifications can enhance selectivity .
Table: Summary of this compound Analogues and Their Selectivity
Analogue | Modification | Target Kinase | Selectivity | Binding Affinity |
---|---|---|---|---|
This compound | None | PKA, PKC | Non-selective | Baseline |
C5(S)-Fluorinated this compound | Fluorination at C5 | PKCε | Improved | Higher than baseline |
Other Fluorinated Analogs | Various fluorinations | Various PKCs | Variable | Depends on structure |
Computational Studies
The use of computational methods such as MD simulations has been pivotal in understanding the dynamics of this compound binding and the effects of structural modifications. These studies have shown that:
Mécanisme D'action
Balanol exerts its effects by binding to the ATP-binding site of serine/threonine kinases, specifically protein kinase A (PKA) and protein kinase C (PKC) . The binding of this compound to these kinases inhibits their activity, thereby disrupting the phosphorylation of target proteins involved in signal transduction pathways . The molecular targets of this compound include the catalytic subunits of PKA and PKC, and its binding mechanism involves interactions with key amino acid residues in the ATP-binding site .
Comparaison Avec Des Composés Similaires
Balanol est unique dans sa structure et ses propriétés de liaison par rapport aux autres inhibiteurs de kinases. Des composés similaires comprennent la staurosporine, l'azépinostatine et l'ophiocordin . Alors que la staurosporine est un inhibiteur de kinase bien connu à large spécificité, le this compound présente une sélectivité plus élevée pour la PKA et la PKC . L'azépinostatine et l'ophiocordin sont structurellement liés au this compound mais diffèrent dans leurs schémas de liaison et leurs affinités de liaison . La structure unique du this compound permet des interactions distinctes avec le site de liaison de l'ATP, ce qui en fait un outil précieux pour étudier l'inhibition des kinases et développer des inhibiteurs sélectifs .
Activité Biologique
Balanol, a fungal metabolite derived from Verticillium balanoides, has garnered significant attention due to its potent inhibitory effects on serine/threonine kinases, particularly Protein Kinase A (PKA) and Protein Kinase C (PKC). This article delves into the biological activity of this compound, exploring its mechanisms of action, structure-activity relationships, and potential therapeutic applications.
This compound functions primarily as an ATP-competitive inhibitor of PKA and PKC. By binding to the ATP-binding site within the catalytic domain of these kinases, this compound prevents the phosphorylation of target substrates, which is essential for the activation of various signaling pathways involved in cell growth and differentiation. The binding affinity of this compound is notably high, with an inhibition constant () reported as low as 4 nM for PKA and varying between 1.6 nM to 742 nM across different PKC isoforms .
Binding Characteristics
The binding mechanism is characterized by nonpolar interactions rather than hydrogen bonding. This compound's structure includes two benzophenone rings that interact with the glycine-rich loop of the kinase, facilitating a rearrangement of catalytic side chains that enhances its inhibitory effect . The flexibility of this compound allows it to adapt to different protein microenvironments, contributing to its selectivity among various kinases.
Structure-Activity Relationships (SAR)
Extensive research has focused on modifying this compound's chemical structure to improve its selectivity and potency. Key findings include:
- Analog Synthesis : Numerous congeners have been synthesized to study the impact of structural modifications on kinase binding. For example, the removal of a hydroxyl group from the benzophenone ring significantly increases selectivity for PKA over PKC .
- Fluorination Effects : Recent studies have shown that introducing fluorine atoms into the this compound structure can enhance selectivity for specific PKC isoforms, particularly PKCε. This modification alters the charge states and binding dynamics within the ATP site .
Modification | Effect on Binding |
---|---|
Hydroxyl group removal | Increased selectivity for PKA |
Fluorination | Improved selectivity for PKCε |
Benzophenone ring flexibility | Enhanced adaptability to kinase environments |
Biological Implications
This compound's ability to inhibit PKA and PKC has significant implications in oncology. PKA is often implicated in tumor promotion, while PKC isoforms can act as either tumor promoters or suppressors depending on the context . Thus, targeting these kinases with this compound or its analogs presents a potential therapeutic strategy in cancer treatment.
Case Studies
- Inhibition Profiles : A study demonstrated that this compound exhibited a potent inhibitory effect on cGMP-dependent protein kinase (PKG), PKA, and various PKC isoforms, with values reflecting its strong competitive inhibition .
- Therapeutic Potential : Research indicates that modifications to enhance selectivity towards specific PKC isoforms could lead to new anticancer drugs that minimize side effects associated with broader kinase inhibition .
Future Directions
Ongoing research aims to further elucidate the precise mechanisms by which this compound and its analogs interact with target kinases. Advanced computational methods such as molecular dynamics simulations are being employed to predict binding affinities and optimize ligand design based on charge states and structural flexibility . The ultimate goal is to develop highly selective inhibitors that can be utilized in clinical settings for targeted cancer therapies.
Propriétés
IUPAC Name |
2-[2,6-dihydroxy-4-[(3R,4R)-3-[(4-hydroxybenzoyl)amino]azepan-4-yl]oxycarbonylbenzoyl]-3-hydroxybenzoic acid | |
---|---|---|
Source | PubChem | |
URL | https://pubchem.ncbi.nlm.nih.gov | |
Description | Data deposited in or computed by PubChem | |
InChI |
InChI=1S/C28H26N2O10/c31-16-8-6-14(7-9-16)26(36)30-18-13-29-10-2-5-22(18)40-28(39)15-11-20(33)24(21(34)12-15)25(35)23-17(27(37)38)3-1-4-19(23)32/h1,3-4,6-9,11-12,18,22,29,31-34H,2,5,10,13H2,(H,30,36)(H,37,38)/t18-,22-/m1/s1 | |
Source | PubChem | |
URL | https://pubchem.ncbi.nlm.nih.gov | |
Description | Data deposited in or computed by PubChem | |
InChI Key |
XYUFCXJZFZPEJD-XMSQKQJNSA-N | |
Source | PubChem | |
URL | https://pubchem.ncbi.nlm.nih.gov | |
Description | Data deposited in or computed by PubChem | |
Canonical SMILES |
C1CC(C(CNC1)NC(=O)C2=CC=C(C=C2)O)OC(=O)C3=CC(=C(C(=C3)O)C(=O)C4=C(C=CC=C4O)C(=O)O)O | |
Source | PubChem | |
URL | https://pubchem.ncbi.nlm.nih.gov | |
Description | Data deposited in or computed by PubChem | |
Isomeric SMILES |
C1C[C@H]([C@@H](CNC1)NC(=O)C2=CC=C(C=C2)O)OC(=O)C3=CC(=C(C(=C3)O)C(=O)C4=C(C=CC=C4O)C(=O)O)O | |
Source | PubChem | |
URL | https://pubchem.ncbi.nlm.nih.gov | |
Description | Data deposited in or computed by PubChem | |
Molecular Formula |
C28H26N2O10 | |
Source | PubChem | |
URL | https://pubchem.ncbi.nlm.nih.gov | |
Description | Data deposited in or computed by PubChem | |
DSSTOX Substance ID |
DTXSID201318143 | |
Record name | (-)-Balanol | |
Source | EPA DSSTox | |
URL | https://comptox.epa.gov/dashboard/DTXSID201318143 | |
Description | DSSTox provides a high quality public chemistry resource for supporting improved predictive toxicology. | |
Molecular Weight |
550.5 g/mol | |
Source | PubChem | |
URL | https://pubchem.ncbi.nlm.nih.gov | |
Description | Data deposited in or computed by PubChem | |
CAS No. |
63590-19-2 | |
Record name | (-)-Balanol | |
Source | CAS Common Chemistry | |
URL | https://commonchemistry.cas.org/detail?cas_rn=63590-19-2 | |
Description | CAS Common Chemistry is an open community resource for accessing chemical information. Nearly 500,000 chemical substances from CAS REGISTRY cover areas of community interest, including common and frequently regulated chemicals, and those relevant to high school and undergraduate chemistry classes. This chemical information, curated by our expert scientists, is provided in alignment with our mission as a division of the American Chemical Society. | |
Explanation | The data from CAS Common Chemistry is provided under a CC-BY-NC 4.0 license, unless otherwise stated. | |
Record name | (-)-Balanol | |
Source | EPA DSSTox | |
URL | https://comptox.epa.gov/dashboard/DTXSID201318143 | |
Description | DSSTox provides a high quality public chemistry resource for supporting improved predictive toxicology. | |
Record name | BALANOL | |
Source | FDA Global Substance Registration System (GSRS) | |
URL | https://gsrs.ncats.nih.gov/ginas/app/beta/substances/O0N0E1MP23 | |
Description | The FDA Global Substance Registration System (GSRS) enables the efficient and accurate exchange of information on what substances are in regulated products. Instead of relying on names, which vary across regulatory domains, countries, and regions, the GSRS knowledge base makes it possible for substances to be defined by standardized, scientific descriptions. | |
Explanation | Unless otherwise noted, the contents of the FDA website (www.fda.gov), both text and graphics, are not copyrighted. They are in the public domain and may be republished, reprinted and otherwise used freely by anyone without the need to obtain permission from FDA. Credit to the U.S. Food and Drug Administration as the source is appreciated but not required. | |
Retrosynthesis Analysis
AI-Powered Synthesis Planning: Our tool employs the Template_relevance Pistachio, Template_relevance Bkms_metabolic, Template_relevance Pistachio_ringbreaker, Template_relevance Reaxys, Template_relevance Reaxys_biocatalysis model, leveraging a vast database of chemical reactions to predict feasible synthetic routes.
One-Step Synthesis Focus: Specifically designed for one-step synthesis, it provides concise and direct routes for your target compounds, streamlining the synthesis process.
Accurate Predictions: Utilizing the extensive PISTACHIO, BKMS_METABOLIC, PISTACHIO_RINGBREAKER, REAXYS, REAXYS_BIOCATALYSIS database, our tool offers high-accuracy predictions, reflecting the latest in chemical research and data.
Strategy Settings
Precursor scoring | Relevance Heuristic |
---|---|
Min. plausibility | 0.01 |
Model | Template_relevance |
Template Set | Pistachio/Bkms_metabolic/Pistachio_ringbreaker/Reaxys/Reaxys_biocatalysis |
Top-N result to add to graph | 6 |
Feasible Synthetic Routes
Avertissement et informations sur les produits de recherche in vitro
Veuillez noter que tous les articles et informations sur les produits présentés sur BenchChem sont destinés uniquement à des fins informatives. Les produits disponibles à l'achat sur BenchChem sont spécifiquement conçus pour des études in vitro, qui sont réalisées en dehors des organismes vivants. Les études in vitro, dérivées du terme latin "in verre", impliquent des expériences réalisées dans des environnements de laboratoire contrôlés à l'aide de cellules ou de tissus. Il est important de noter que ces produits ne sont pas classés comme médicaments et n'ont pas reçu l'approbation de la FDA pour la prévention, le traitement ou la guérison de toute condition médicale, affection ou maladie. Nous devons souligner que toute forme d'introduction corporelle de ces produits chez les humains ou les animaux est strictement interdite par la loi. Il est essentiel de respecter ces directives pour assurer la conformité aux normes légales et éthiques en matière de recherche et d'expérimentation.