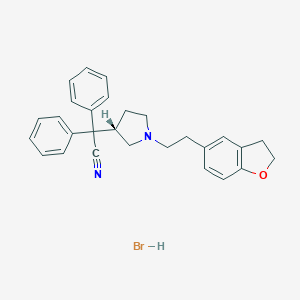
2DP6AVY7RB
- Cliquez sur DEMANDE RAPIDE pour recevoir un devis de notre équipe d'experts.
- Avec des produits de qualité à un prix COMPÉTITIF, vous pouvez vous concentrer davantage sur votre recherche.
Vue d'ensemble
Description
(3S)-1-[2-(2,3-Dihydro-5-benzofuranyl)ethyl]-alpha,alpha-diphenyl-3-pyrrolidineacetonitrile Hydrobromide, also known as (3S)-1-[2-(2,3-Dihydro-5-benzofuranyl)ethyl]-alpha,alpha-diphenyl-3-pyrrolidineacetonitrile Hydrobromide, is a useful research compound. Its molecular formula is C28H29BrN2O and its molecular weight is 489.4 g/mol. The purity is usually 95%.
BenchChem offers high-quality (3S)-1-[2-(2,3-Dihydro-5-benzofuranyl)ethyl]-alpha,alpha-diphenyl-3-pyrrolidineacetonitrile Hydrobromide suitable for many research applications. Different packaging options are available to accommodate customers' requirements. Please inquire for more information about (3S)-1-[2-(2,3-Dihydro-5-benzofuranyl)ethyl]-alpha,alpha-diphenyl-3-pyrrolidineacetonitrile Hydrobromide including the price, delivery time, and more detailed information at info@benchchem.com.
Applications De Recherche Scientifique
Développement d'un gel de bromhydrate de darifénacine à base de nanovecteurs
Le bromhydrate de darifénacine, un médicament de classe II de la BCS, présente une faible biodisponibilité en raison d'un métabolisme important de premier passage . Une étude a examiné une voie de délivrance de médicaments alternative en développant un gel transdermique à base de microémulsion nanométrique pour la prise en charge de la vessie hyperactive . Les avantages obtenus pourraient se traduire par une biodisponibilité accrue et une réduction de la dose .
Nanoparticules cubiques de cristal liquide auto-assemblées
Une autre étude a mis au point des nanoparticules cubiques de cristal liquide (LCCN) auto-assemblées chargées de darifénacine pour une approche de libération prolongée afin de contrôler la vessie hyperactive pendant la nuit . La formule optimisée présentait une petite taille de particules, une bonne homogénéité et un potentiel zêta ainsi qu'un profil de libération in vitro contrôlé et une perméation ex vivo à travers l'intestin de lapin .
Synthèse/isolement des sous-produits du bromhydrate de darifénacine
Le spectre de masse a montré le pic d'ion moléculaire protoné à m/z 572 unités de masse atomique, similaire à celui du dimère-1 . Cela suggère que le bromhydrate de darifénacine peut être utilisé dans la synthèse/l'isolement de ses sous-produits .
Préparation de transporteurs lipidiques nanostructurés chargés de bromhydrate de darifénacine
Des transporteurs lipidiques nanostructurés (NLC) chargés de bromhydrate de darifénacine ont été formulés par sonication d'émulsification en utilisant différents rapports de lipide solide à lipide liquide
Mécanisme D'action
Target of Action
Darifenacin nitrile hydrobromide, also known as 2DP6AVY7RB, primarily targets the M3 muscarinic acetylcholine receptors . These receptors play a crucial role in mediating bladder muscle contractions .
Mode of Action
The compound acts as a competitive antagonist of the M3 muscarinic acetylcholine receptors . By binding to these receptors, it inhibits their activation, thereby reducing bladder muscle contractions . This blockage decreases the urgency to urinate .
Biochemical Pathways
The primary biochemical pathway affected by this compound is the muscarinic signaling pathway . By blocking the M3 receptors, darifenacin nitrile hydrobromide disrupts the normal signaling process, leading to reduced bladder muscle contractions .
Pharmacokinetics
The pharmacokinetics of darifenacin involve several key aspects:
- Absorption : After oral administration, darifenacin is well absorbed from the gastrointestinal tract .
- Distribution : It is highly protein-bound (98%), primarily to α1-acid glycoprotein .
- Metabolism : Darifenacin is subject to extensive hepatic metabolism, mediated by hepatic cytochrome P450 2D6 and 3A4 .
- Excretion : Only about 3% of unchanged drug is excreted in urine and feces .
Result of Action
The primary molecular effect of darifenacin nitrile hydrobromide’s action is the reduction of bladder muscle contractions . On a cellular level, this results in decreased urgency to urinate .
Action Environment
The action, efficacy, and stability of darifenacin nitrile hydrobromide can be influenced by various environmental factors. For instance, the presence of other drugs can affect its metabolism and excretion . Additionally, conditions such as hepatic impairment can increase plasma concentrations of the drug .
Analyse Biochimique
Biochemical Properties
It is known that the compound is subject to extensive hepatic metabolism . The main metabolic routes are monohydroxylation in the dihydrobenzfuran ring, dihydrobenzfuran ring opening, and N-dealkylation of the pyrrolidine nitrogen . These reactions are mediated by hepatic cytochrome P450 2D6 and 3A4 .
Cellular Effects
It is known that darifenacin, a related compound, blocks M3 muscarinic acetylcholine receptors, which mediate bladder muscle contractions . This block reduces the urgency to urinate .
Molecular Mechanism
It is known that darifenacin, a related compound, works by blocking M3 muscarinic acetylcholine receptors . This block reduces the urgency to urinate .
Propriétés
IUPAC Name |
2-[(3S)-1-[2-(2,3-dihydro-1-benzofuran-5-yl)ethyl]pyrrolidin-3-yl]-2,2-diphenylacetonitrile;hydrobromide |
Source
|
---|---|---|
Source | PubChem | |
URL | https://pubchem.ncbi.nlm.nih.gov | |
Description | Data deposited in or computed by PubChem | |
InChI |
InChI=1S/C28H28N2O.BrH/c29-21-28(24-7-3-1-4-8-24,25-9-5-2-6-10-25)26-14-17-30(20-26)16-13-22-11-12-27-23(19-22)15-18-31-27;/h1-12,19,26H,13-18,20H2;1H/t26-;/m1./s1 |
Source
|
Source | PubChem | |
URL | https://pubchem.ncbi.nlm.nih.gov | |
Description | Data deposited in or computed by PubChem | |
InChI Key |
CIQVSKIIOIPTSE-UFTMZEDQSA-N |
Source
|
Source | PubChem | |
URL | https://pubchem.ncbi.nlm.nih.gov | |
Description | Data deposited in or computed by PubChem | |
Canonical SMILES |
C1CN(CC1C(C#N)(C2=CC=CC=C2)C3=CC=CC=C3)CCC4=CC5=C(C=C4)OCC5.Br |
Source
|
Source | PubChem | |
URL | https://pubchem.ncbi.nlm.nih.gov | |
Description | Data deposited in or computed by PubChem | |
Isomeric SMILES |
C1CN(C[C@@H]1C(C#N)(C2=CC=CC=C2)C3=CC=CC=C3)CCC4=CC5=C(C=C4)OCC5.Br |
Source
|
Source | PubChem | |
URL | https://pubchem.ncbi.nlm.nih.gov | |
Description | Data deposited in or computed by PubChem | |
Molecular Formula |
C28H29BrN2O |
Source
|
Source | PubChem | |
URL | https://pubchem.ncbi.nlm.nih.gov | |
Description | Data deposited in or computed by PubChem | |
Molecular Weight |
489.4 g/mol |
Source
|
Source | PubChem | |
URL | https://pubchem.ncbi.nlm.nih.gov | |
Description | Data deposited in or computed by PubChem | |
CAS No. |
608127-89-5 |
Source
|
Record name | Darifenacin nitrile hydrobromide | |
Source | ChemIDplus | |
URL | https://pubchem.ncbi.nlm.nih.gov/substance/?source=chemidplus&sourceid=0608127895 | |
Description | ChemIDplus is a free, web search system that provides access to the structure and nomenclature authority files used for the identification of chemical substances cited in National Library of Medicine (NLM) databases, including the TOXNET system. | |
Record name | DARIFENACIN NITRILE HYDROBROMIDE | |
Source | FDA Global Substance Registration System (GSRS) | |
URL | https://gsrs.ncats.nih.gov/ginas/app/beta/substances/2DP6AVY7RB | |
Description | The FDA Global Substance Registration System (GSRS) enables the efficient and accurate exchange of information on what substances are in regulated products. Instead of relying on names, which vary across regulatory domains, countries, and regions, the GSRS knowledge base makes it possible for substances to be defined by standardized, scientific descriptions. | |
Explanation | Unless otherwise noted, the contents of the FDA website (www.fda.gov), both text and graphics, are not copyrighted. They are in the public domain and may be republished, reprinted and otherwise used freely by anyone without the need to obtain permission from FDA. Credit to the U.S. Food and Drug Administration as the source is appreciated but not required. | |
Avertissement et informations sur les produits de recherche in vitro
Veuillez noter que tous les articles et informations sur les produits présentés sur BenchChem sont destinés uniquement à des fins informatives. Les produits disponibles à l'achat sur BenchChem sont spécifiquement conçus pour des études in vitro, qui sont réalisées en dehors des organismes vivants. Les études in vitro, dérivées du terme latin "in verre", impliquent des expériences réalisées dans des environnements de laboratoire contrôlés à l'aide de cellules ou de tissus. Il est important de noter que ces produits ne sont pas classés comme médicaments et n'ont pas reçu l'approbation de la FDA pour la prévention, le traitement ou la guérison de toute condition médicale, affection ou maladie. Nous devons souligner que toute forme d'introduction corporelle de ces produits chez les humains ou les animaux est strictement interdite par la loi. Il est essentiel de respecter ces directives pour assurer la conformité aux normes légales et éthiques en matière de recherche et d'expérimentation.