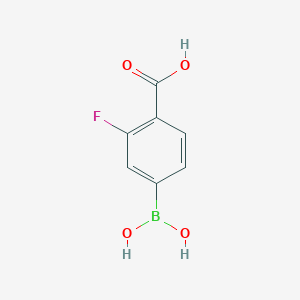
Acide 4-carboxy-3-fluorophénylboronique
Vue d'ensemble
Description
Synthesis Analysis
The synthesis of derivatives similar to 4-carboxy-3-fluorophenylboronic acid often involves halogen-lithium exchange reactions, followed by the addition of trimethyl borate and subsequent hydrolysis. For instance, the synthesis of amino-3-fluorophenyl boronic acid was achieved through such a methodology, with a reported yield of 47% (Das et al., 2003). This process highlights the general approach to synthesizing boronic acid derivatives with specific substituents on the phenyl ring.
Molecular Structure Analysis
The molecular structure of boronic acid derivatives, including those with carboxy and fluorine substituents, has been extensively studied. For example, solid-state structures of 4-carboxyphenylboronic acid and its hydrates have been reported, demonstrating the impact of hydration on molecular conformation and intermolecular interactions. These studies reveal that hydrogen bonding plays a significant role in the crystal packing of these compounds, leading to diverse solid-state architectures (SeethaLekshmi & Pedireddi, 2007).
Chemical Reactions and Properties
Boronic acids, including 4-carboxy-3-fluorophenylboronic acid, are pivotal in organic synthesis, particularly in Suzuki coupling reactions. These reactions are essential for forming carbon-carbon bonds, thereby constructing complex molecular frameworks. The presence of fluorine and carboxy groups influences the reactivity and stability of the boronic acid, affecting its behavior in these coupling reactions.
Physical Properties Analysis
The physical properties of boronic acid derivatives are influenced by their molecular structure. For example, the hydration state of 4-carboxyphenylboronic acid affects its crystallization and, consequently, its physical appearance. The analysis of solid-state structures provides insights into the impact of substituents on the physical properties of these compounds.
Chemical Properties Analysis
The chemical properties of 4-carboxy-3-fluorophenylboronic acid are characterized by its reactivity in various chemical reactions, including its role as a catalyst or reactant in organic synthesis. The ortho-substituent on phenylboronic acids, such as in the case of 2,4-bis(trifluoromethyl)phenylboronic acid, has been shown to catalyze dehydrative condensation reactions between carboxylic acids and amines, indicating the influence of substituent positioning on chemical reactivity (Wang, Lu, & Ishihara, 2018).
Applications De Recherche Scientifique
Applications biomédicales
Les conjugués de chitosane fonctionnalisés par l'acide phénylboronique ont été utilisés pour une variété d'applications biomédicales . Ces conjugués agissent comme des polymères sensibles au glucose, ce qui permet une libération d'insuline autorégulée dans le traitement du diabète . Ils agissent également comme agents de diagnostic .
Cicatrisation
Les conjugués de chitosane fonctionnalisés par l'acide phénylboronique ont également été utilisés pour la cicatrisation . La conjugaison de ces deux entités a montré qu'elle présentait des propriétés qui peuvent être exploitées pour cette application .
Ciblage tumoral
Les conjugués de chitosane fonctionnalisés par l'acide phénylboronique ont été utilisés pour le ciblage tumoral . Cette application utilise les propriétés spécifiques de ces conjugués .
Réactions de couplage
L'acide 4-fluorophénylboronique peut être utilisé comme réactif dans des réactions de couplage avec des tétrafluoroborates d'arènediazonium, des sels d'iodonium et des iodanes . Cette application est particulièrement pertinente dans le domaine de la chimie synthétique .
6. Synthèse de nouveaux terphényles biologiquement actifs L'acide 4-fluorophénylboronique est utilisé pour fabriquer de nouveaux terphényles biologiquement actifs
Safety and Hazards
The safety information for 4-Carboxy-3-fluorophenylboronic acid includes hazard statements H315, H319, H335, indicating that it can cause skin irritation, serious eye irritation, and may cause respiratory irritation . Precautionary measures include avoiding breathing dust/fume/gas/mist/vapors/spray, washing hands thoroughly after handling, and wearing protective gloves/protective clothing/eye protection/face protection .
Orientations Futures
4-Carboxy-3-fluorophenylboronic acid has been used in the synthesis of glucose-responsive 4-carboxy-3-fluorophenylboronic acid-grafted ε-polylysine (CFPBA- g -PL) for glucose-responsive insulin delivery via transdermal microneedles . This represents a promising direction for the application of this compound in the field of diabetes management .
Mécanisme D'action
Target of Action
4-Carboxy-3-fluorophenylboronic acid is primarily used as a reagent in the discovery of novel azaindole-based series as potent AXL kinase inhibitors . AXL kinase is a receptor tyrosine kinase that plays essential roles in various cellular processes, including proliferation, survival, and migration .
Mode of Action
The compound interacts with its targets through Suzuki–Miyaura (SM) cross-coupling, a widely-applied transition metal catalysed carbon–carbon bond forming reaction . This process involves the transfer of formally nucleophilic organic groups from boron to palladium .
Biochemical Pathways
It’s known that the compound plays a role in the synthesis of novel azaindole-based series, which can inhibit axl kinase . This suggests that the compound may affect pathways involving AXL kinase.
Pharmacokinetics
The pharmacokinetics of 4-Carboxy-3-fluorophenylboronic acid-grafted ε-polylysine (CFPBA-g-PL) have been studied . CFPBA-g-PL has low cytotoxicity, good hemocompatibility, and no tissue reaction . .
Result of Action
The molecular and cellular effects of 4-Carboxy-3-fluorophenylboronic acid’s action are largely dependent on its role in the synthesis of novel azaindole-based series . These series can inhibit AXL kinase, potentially affecting cellular processes such as proliferation, survival, and migration .
Action Environment
The action, efficacy, and stability of 4-Carboxy-3-fluorophenylboronic acid can be influenced by various environmental factors. For instance, the compound is used under exceptionally mild and functional group tolerant reaction conditions in Suzuki–Miyaura coupling . Additionally, it should be stored in an inert atmosphere at 2-8°C .
Propriétés
IUPAC Name |
4-borono-2-fluorobenzoic acid | |
---|---|---|
Source | PubChem | |
URL | https://pubchem.ncbi.nlm.nih.gov | |
Description | Data deposited in or computed by PubChem | |
InChI |
InChI=1S/C7H6BFO4/c9-6-3-4(8(12)13)1-2-5(6)7(10)11/h1-3,12-13H,(H,10,11) | |
Source | PubChem | |
URL | https://pubchem.ncbi.nlm.nih.gov | |
Description | Data deposited in or computed by PubChem | |
InChI Key |
CZDWJVSOQOMYGC-UHFFFAOYSA-N | |
Source | PubChem | |
URL | https://pubchem.ncbi.nlm.nih.gov | |
Description | Data deposited in or computed by PubChem | |
Canonical SMILES |
B(C1=CC(=C(C=C1)C(=O)O)F)(O)O | |
Source | PubChem | |
URL | https://pubchem.ncbi.nlm.nih.gov | |
Description | Data deposited in or computed by PubChem | |
Molecular Formula |
C7H6BFO4 | |
Source | PubChem | |
URL | https://pubchem.ncbi.nlm.nih.gov | |
Description | Data deposited in or computed by PubChem | |
DSSTOX Substance ID |
DTXSID90376807 | |
Record name | 4-Carboxy-3-fluorophenylboronic acid | |
Source | EPA DSSTox | |
URL | https://comptox.epa.gov/dashboard/DTXSID90376807 | |
Description | DSSTox provides a high quality public chemistry resource for supporting improved predictive toxicology. | |
Molecular Weight |
183.93 g/mol | |
Source | PubChem | |
URL | https://pubchem.ncbi.nlm.nih.gov | |
Description | Data deposited in or computed by PubChem | |
CAS RN |
120153-08-4 | |
Record name | 4-Borono-2-fluorobenzoic acid | |
Source | ChemIDplus | |
URL | https://pubchem.ncbi.nlm.nih.gov/substance/?source=chemidplus&sourceid=0120153084 | |
Description | ChemIDplus is a free, web search system that provides access to the structure and nomenclature authority files used for the identification of chemical substances cited in National Library of Medicine (NLM) databases, including the TOXNET system. | |
Record name | 4-Carboxy-3-fluorophenylboronic acid | |
Source | EPA DSSTox | |
URL | https://comptox.epa.gov/dashboard/DTXSID90376807 | |
Description | DSSTox provides a high quality public chemistry resource for supporting improved predictive toxicology. | |
Record name | 4-Carboxy-3-fluorobenzeneboronic acid | |
Source | European Chemicals Agency (ECHA) | |
URL | https://echa.europa.eu/information-on-chemicals | |
Description | The European Chemicals Agency (ECHA) is an agency of the European Union which is the driving force among regulatory authorities in implementing the EU's groundbreaking chemicals legislation for the benefit of human health and the environment as well as for innovation and competitiveness. | |
Explanation | Use of the information, documents and data from the ECHA website is subject to the terms and conditions of this Legal Notice, and subject to other binding limitations provided for under applicable law, the information, documents and data made available on the ECHA website may be reproduced, distributed and/or used, totally or in part, for non-commercial purposes provided that ECHA is acknowledged as the source: "Source: European Chemicals Agency, http://echa.europa.eu/". Such acknowledgement must be included in each copy of the material. ECHA permits and encourages organisations and individuals to create links to the ECHA website under the following cumulative conditions: Links can only be made to webpages that provide a link to the Legal Notice page. | |
Record name | 4-Borono-2-fluorobenzoic acid | |
Source | FDA Global Substance Registration System (GSRS) | |
URL | https://gsrs.ncats.nih.gov/ginas/app/beta/substances/MT2PH75ZYW | |
Description | The FDA Global Substance Registration System (GSRS) enables the efficient and accurate exchange of information on what substances are in regulated products. Instead of relying on names, which vary across regulatory domains, countries, and regions, the GSRS knowledge base makes it possible for substances to be defined by standardized, scientific descriptions. | |
Explanation | Unless otherwise noted, the contents of the FDA website (www.fda.gov), both text and graphics, are not copyrighted. They are in the public domain and may be republished, reprinted and otherwise used freely by anyone without the need to obtain permission from FDA. Credit to the U.S. Food and Drug Administration as the source is appreciated but not required. | |
Retrosynthesis Analysis
AI-Powered Synthesis Planning: Our tool employs the Template_relevance Pistachio, Template_relevance Bkms_metabolic, Template_relevance Pistachio_ringbreaker, Template_relevance Reaxys, Template_relevance Reaxys_biocatalysis model, leveraging a vast database of chemical reactions to predict feasible synthetic routes.
One-Step Synthesis Focus: Specifically designed for one-step synthesis, it provides concise and direct routes for your target compounds, streamlining the synthesis process.
Accurate Predictions: Utilizing the extensive PISTACHIO, BKMS_METABOLIC, PISTACHIO_RINGBREAKER, REAXYS, REAXYS_BIOCATALYSIS database, our tool offers high-accuracy predictions, reflecting the latest in chemical research and data.
Strategy Settings
Precursor scoring | Relevance Heuristic |
---|---|
Min. plausibility | 0.01 |
Model | Template_relevance |
Template Set | Pistachio/Bkms_metabolic/Pistachio_ringbreaker/Reaxys/Reaxys_biocatalysis |
Top-N result to add to graph | 6 |
Feasible Synthetic Routes
Q & A
Q1: How does 4-Carboxy-3-fluorophenylboronic acid interact with glucose and what are the downstream effects?
A1: 4-Carboxy-3-fluorophenylboronic acid (FPBA) reversibly binds to glucose through its boronic acid moiety, forming a cyclic ester. [, , , , , , , ] This binding event triggers a change in the physicochemical properties of FPBA-containing materials. For example, it can lead to a shift in the material's charge from neutral to negative, increase its hydrophilicity, or induce disassembly of self-assembled structures. [, , , ] These changes can be harnessed for glucose-responsive drug release.
Q2: What is the impact of the fluorine atom in 4-Carboxy-3-fluorophenylboronic acid compared to the non-fluorinated analog?
A2: The fluorine atom in FPBA increases its acidity compared to 4-carboxyphenylboronic acid (PBA). [, ] This results in a lower pKa value for FPBA, enabling it to bind glucose more effectively at physiological pH. [] Consequently, FPBA-based systems often exhibit enhanced glucose sensitivity and responsiveness compared to PBA-based counterparts. [, ]
Q3: What are some examples of how 4-Carboxy-3-fluorophenylboronic acid has been incorporated into glucose-responsive drug delivery systems?
A3: FPBA has been incorporated into various drug delivery systems, including:
- Polyplex micelles: FPBA-modified polyplex micelles have been developed for both gene [, ] and peptide delivery. [] These micelles exhibit glucose-responsive changes in their core crosslinking, leading to controlled release of the therapeutic payload.
- Nanoparticles: FPBA-modified nanoparticles have been designed for insulin delivery. [, , ] The nanoparticles release insulin in response to elevated glucose levels, mimicking the function of pancreatic beta cells.
- Microneedles: Biodegradable microneedles encapsulating FPBA-modified particles have been explored for transdermal insulin delivery. [] These microneedles offer a minimally invasive approach for glucose-responsive insulin administration.
Q4: What are the potential advantages of using 4-Carboxy-3-fluorophenylboronic acid in glucose-responsive drug delivery systems?
A4: FPBA offers several advantages for glucose-responsive drug delivery, including:
- Biocompatibility: FPBA-modified materials have demonstrated good biocompatibility in several studies. [, , ]
- Tunable Responsiveness: The glucose sensitivity and response kinetics of FPBA-based systems can be fine-tuned by adjusting factors like the degree of FPBA modification and the overall material composition. [, , ]
- Versatility: FPBA can be conjugated to various polymers, lipids, and nanoparticles, enabling the development of diverse glucose-responsive drug delivery platforms. [, , , , , ]
Q5: What are the limitations or challenges associated with using 4-Carboxy-3-fluorophenylboronic acid in drug delivery systems?
A5: Some challenges associated with using FPBA include:
- Long-term stability: The long-term stability of FPBA-modified materials and their potential for degradation in vivo requires further investigation. []
- Immunogenicity: The potential immunogenicity of FPBA-containing systems, particularly with repeated administration, needs to be carefully assessed. []
Q6: What are the future directions for research on 4-Carboxy-3-fluorophenylboronic acid in drug delivery?
A6: Future research could focus on:
Avertissement et informations sur les produits de recherche in vitro
Veuillez noter que tous les articles et informations sur les produits présentés sur BenchChem sont destinés uniquement à des fins informatives. Les produits disponibles à l'achat sur BenchChem sont spécifiquement conçus pour des études in vitro, qui sont réalisées en dehors des organismes vivants. Les études in vitro, dérivées du terme latin "in verre", impliquent des expériences réalisées dans des environnements de laboratoire contrôlés à l'aide de cellules ou de tissus. Il est important de noter que ces produits ne sont pas classés comme médicaments et n'ont pas reçu l'approbation de la FDA pour la prévention, le traitement ou la guérison de toute condition médicale, affection ou maladie. Nous devons souligner que toute forme d'introduction corporelle de ces produits chez les humains ou les animaux est strictement interdite par la loi. Il est essentiel de respecter ces directives pour assurer la conformité aux normes légales et éthiques en matière de recherche et d'expérimentation.