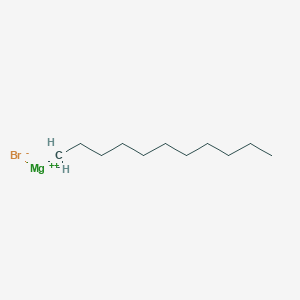
Undecylmagnesium bromide
Vue d'ensemble
Description
Undecylmagnesium bromide is a chemical compound with the formula C11H23BrMg. It has a molecular weight of 259.5117 .
Synthesis Analysis
The synthesis of compounds similar to undecylmagnesium bromide often involves the use of Grignard reagents, which are formed by the reaction of an alkyl or aryl halide with magnesium in an ether solution . The process of retrosynthetic analysis, a technique frequently used in organic synthesis, can be applied to identify the precursors needed for the synthesis .Molecular Structure Analysis
The molecular structure of undecylmagnesium bromide can be analyzed using various techniques. For instance, mass spectrometry can be used to interpret the fragmentation pattern of the mass spectrum of a compound . Additionally, the molecular formula of a compound can be determined using MS and combustion analysis .Chemical Reactions Analysis
Undecylmagnesium bromide can participate in various chemical reactions. For example, it has been used in the reaction with 4-chloropyridine in tetrahydrofuran (THF) at -78 C. Electroanalytical tools can be utilized to investigate redox-active intermediates involved in these reactions .Physical And Chemical Properties Analysis
The physical and chemical properties of undecylmagnesium bromide can be analyzed using various techniques. For instance, the properties of polymeric materials can be screened in high throughput . Additionally, the behavior of magnesium bromide under high pressure has been studied .Applications De Recherche Scientifique
Synthesis of Biocompatible Nanoparticles
Undecylmagnesium bromide can be used in the synthesis of magnesium oxide (MgO) nanoparticles . These nanoparticles have excellent biocompatibility, stability, and diverse biomedical uses, such as antimicrobial, antioxidant, anticancer, and antidiabetic properties, as well as tissue engineering, bioimaging, and drug delivery applications .
Preparation of 2,4,5-Trifluorobenzoic Acid
Undecylmagnesium bromide can be used in a continuous flow microreactor system for the preparation of 2,4,5-trifluorobenzoic acid via a two-step reaction involving halogen–magnesium exchange and carboxylation . This acid is a valuable synthetic intermediate with important applications in the pharmaceutical industry .
Safety and Hazards
Orientations Futures
The future directions of research involving undecylmagnesium bromide could involve its use in sustainable economic development. For instance, catalytic chemistry, which involves compounds like undecylmagnesium bromide, plays a crucial role in the synthesis of chemicals and materials, energy production, and pollution abatement .
Propriétés
IUPAC Name |
magnesium;undecane;bromide | |
---|---|---|
Source | PubChem | |
URL | https://pubchem.ncbi.nlm.nih.gov | |
Description | Data deposited in or computed by PubChem | |
InChI |
InChI=1S/C11H23.BrH.Mg/c1-3-5-7-9-11-10-8-6-4-2;;/h1,3-11H2,2H3;1H;/q-1;;+2/p-1 | |
Source | PubChem | |
URL | https://pubchem.ncbi.nlm.nih.gov | |
Description | Data deposited in or computed by PubChem | |
InChI Key |
BMSODDZZHKPPOK-UHFFFAOYSA-M | |
Source | PubChem | |
URL | https://pubchem.ncbi.nlm.nih.gov | |
Description | Data deposited in or computed by PubChem | |
Canonical SMILES |
CCCCCCCCCC[CH2-].[Mg+2].[Br-] | |
Source | PubChem | |
URL | https://pubchem.ncbi.nlm.nih.gov | |
Description | Data deposited in or computed by PubChem | |
Molecular Formula |
C11H23BrMg | |
Source | PubChem | |
URL | https://pubchem.ncbi.nlm.nih.gov | |
Description | Data deposited in or computed by PubChem | |
Molecular Weight |
259.51 g/mol | |
Source | PubChem | |
URL | https://pubchem.ncbi.nlm.nih.gov | |
Description | Data deposited in or computed by PubChem | |
Product Name |
Undecylmagnesium bromide |
Retrosynthesis Analysis
AI-Powered Synthesis Planning: Our tool employs the Template_relevance Pistachio, Template_relevance Bkms_metabolic, Template_relevance Pistachio_ringbreaker, Template_relevance Reaxys, Template_relevance Reaxys_biocatalysis model, leveraging a vast database of chemical reactions to predict feasible synthetic routes.
One-Step Synthesis Focus: Specifically designed for one-step synthesis, it provides concise and direct routes for your target compounds, streamlining the synthesis process.
Accurate Predictions: Utilizing the extensive PISTACHIO, BKMS_METABOLIC, PISTACHIO_RINGBREAKER, REAXYS, REAXYS_BIOCATALYSIS database, our tool offers high-accuracy predictions, reflecting the latest in chemical research and data.
Strategy Settings
Precursor scoring | Relevance Heuristic |
---|---|
Min. plausibility | 0.01 |
Model | Template_relevance |
Template Set | Pistachio/Bkms_metabolic/Pistachio_ringbreaker/Reaxys/Reaxys_biocatalysis |
Top-N result to add to graph | 6 |
Feasible Synthetic Routes
Avertissement et informations sur les produits de recherche in vitro
Veuillez noter que tous les articles et informations sur les produits présentés sur BenchChem sont destinés uniquement à des fins informatives. Les produits disponibles à l'achat sur BenchChem sont spécifiquement conçus pour des études in vitro, qui sont réalisées en dehors des organismes vivants. Les études in vitro, dérivées du terme latin "in verre", impliquent des expériences réalisées dans des environnements de laboratoire contrôlés à l'aide de cellules ou de tissus. Il est important de noter que ces produits ne sont pas classés comme médicaments et n'ont pas reçu l'approbation de la FDA pour la prévention, le traitement ou la guérison de toute condition médicale, affection ou maladie. Nous devons souligner que toute forme d'introduction corporelle de ces produits chez les humains ou les animaux est strictement interdite par la loi. Il est essentiel de respecter ces directives pour assurer la conformité aux normes légales et éthiques en matière de recherche et d'expérimentation.