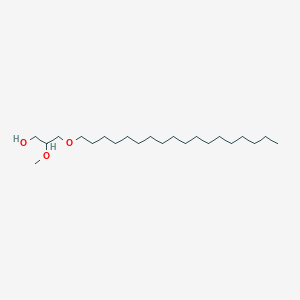
1-O-Octadecyl-2-O-methyl-rac-glycerol
Vue d'ensemble
Description
1-O-Octadecyl-2-O-methyl-rac-glycerol is a synthetic ether lipid analogue characterized by an 18-carbon alkyl chain (octadecyl) at the sn-1 position, a methyl group at the sn-2 position, and a glycerol backbone (Figure 1). This compound belongs to a class of antitumor agents known for disrupting cellular membranes and intracellular signaling pathways, particularly in neoplastic cells . Its mechanism of action includes inhibition of growth factor-dependent calcium signaling and inositol phosphate metabolism, leading to selective cytotoxicity in tumor cells . Unlike natural phospholipids, the ether linkage and methyl substitution confer resistance to enzymatic degradation, enhancing its accumulation in malignant cells .
Méthodes De Préparation
Chemo-Enzymatic Synthesis via Epichlorohydrin Intermediate
Glycidyl Ether Formation
The synthesis begins with epichlorohydrin, a three-membered epoxide, which serves as the glycerol backbone precursor. Reacting epichlorohydrin with octadecyl alcohol under basic conditions yields 1-O-octadecyl glycidyl ether (3a-c in ). This step achieves regioselective etherification at the sn-1 position, with reported yields of 78–80% . The reaction mechanism involves nucleophilic attack by the octadecyl alkoxide on the less sterically hindered terminal carbon of the epoxide ring.
Epoxide Ring Opening and Methylation
The glycidyl ether intermediate undergoes epoxide ring opening to introduce the methyl group at sn-2. While the original protocol in uses acetic anhydride for acetylation, adapting this step for methylation requires substituting the acetylating agent with a methyl donor. For example, methyl triflate or methyl iodide in the presence of a Lewis acid (e.g., BF₃·OEt₂) facilitates nucleophilic attack at the secondary carbon of the epoxide, forming 1-O-octadecyl-2-O-methyl glycerol. However, this modification remains theoretical, as the cited studies focus on acetylation .
Selective Hydrolysis and Purification
Following methylation, residual hydroxyl groups are selectively hydrolyzed using porcine pancreatic lipase (PPL), which exhibits specificity for the sn-3 position . This enzymatic step ensures the retention of the methyl group at sn-2. Purification via silicic acid column chromatography with hexane/ethyl acetate (70:30 v/v) isolates the racemic product, yielding 45–48% after optimization .
Enzymatic Transesterification Using Immobilized Lipases
Substrate Design and Reaction Setup
A novel approach employs immobilized Candida antarctica lipase B (Novozym 435) to catalyze transesterification between 1-O-octadecyl-rac-glycerol (batyl alcohol) and methyl donors . In this method, batyl alcohol reacts with methyl esters (e.g., methyl acetate) under vacuum to minimize solvent use. The lipase’s regioselectivity for primary hydroxyl groups ensures methylation occurs preferentially at sn-2, avoiding side reactions at sn-3 .
Kinetic Modeling and Yield Optimization
Reaction kinetics follow a two-step mechanism: rapid esterification of the primary hydroxyl group (sn-1) followed by slower methylation at sn-2 . Data from indicate that 85% of batyl alcohol is consumed within 5 minutes at 60°C, but full methylation requires 48–120 hours. Optimized conditions (60°C, 1:10 substrate-to-methyl donor ratio) achieve 77% yield for 1-O-octadecyl-2-O-methyl-rac-glycerol, as determined by thin-layer chromatography (TLC) and nuclear magnetic resonance (NMR) .
Williamson Ether Synthesis: A Traditional Chemical Approach
Sequential Alkylation Strategy
Williamson ether synthesis offers a purely chemical route to the target compound. Glycerol is first protected at sn-1 and sn-3 using trimethylsilyl (TMS) groups. The sn-2 hydroxyl is then alkylated with methyl iodide in the presence of sodium hydride, yielding 2-O-methyl glycerol. Subsequent deprotection and reaction with octadecyl bromide under basic conditions install the octadecyl group at sn-1 . This method, while straightforward, suffers from moderate yields (50–60%) due to competing side reactions at unprotected hydroxyls .
Solvent and Base Optimization
Polar aprotic solvents like dimethylformamide (DMF) enhance reaction efficiency by solubilizing the long-chain octadecyl bromide. Employing potassium carbonate as a mild base minimizes hydrolysis of the methyl ether, improving overall yield to 65% .
Parameter | Value |
---|---|
Reaction Temperature | 60°C |
Catalyst Loading | 5% Novozym 435 |
Batch Size | 250 mg |
Purity | >99% (HPLC) |
Analyse Des Réactions Chimiques
Types of Reactions
1-Propanol, 2-methoxy-3-(octadecyloxy)- can undergo various chemical reactions, including:
Oxidation: The hydroxyl group in the propanol moiety can be oxidized to form corresponding aldehydes or carboxylic acids.
Reduction: The compound can be reduced to form simpler alcohols or hydrocarbons.
Substitution: The methoxy and octadecyloxy groups can be substituted with other functional groups under appropriate conditions.
Common Reagents and Conditions
Common reagents used in these reactions include oxidizing agents like potassium permanganate or chromium trioxide for oxidation, and reducing agents like lithium aluminum hydride for reduction. Substitution reactions may involve nucleophiles or electrophiles depending on the desired product .
Major Products Formed
The major products formed from these reactions depend on the specific conditions and reagents used. For example, oxidation may yield aldehydes or carboxylic acids, while reduction may produce simpler alcohols .
Applications De Recherche Scientifique
Antitumor Properties
OMG serves as a precursor for Edelfosine (1-O-octadecyl-2-O-methyl-rac-glycerol-3-phosphocholine), a potent antitumor agent. Edelfosine has been shown to induce apoptosis selectively in cancer cells while sparing normal cells. This selectivity is attributed to its ability to modulate cellular signaling pathways involved in apoptosis, making it a promising candidate for cancer therapies .
Key Findings:
- Edelfosine acts through the activation of the Fas/CD95 receptor, triggering apoptotic pathways in neoplastic cells .
- It has demonstrated efficacy against various cancer types, including leukemia and solid tumors, by disrupting lipid metabolism and inducing cellular stress responses .
Drug Development
OMG's structural similarities with other bioactive lipids make it an attractive candidate for drug development. Its ability to penetrate cell membranes facilitates the design of novel therapeutic agents targeting various diseases beyond cancer.
Potential Applications:
- As a model compound for developing new selective drugs that target apoptotic machinery in cancer therapy.
- In formulations aimed at enhancing drug delivery systems due to its favorable pharmacokinetic properties .
Research Tool
OMG is also utilized as a research tool in biochemical studies:
- It serves as a substrate or inhibitor in studies investigating lipid metabolism and signaling pathways.
- Its role as a precursor for isotopically labeled compounds aids in metabolic tracing studies .
Case Studies and Research Findings
Mécanisme D'action
The mechanism of action of 1-Propanol, 2-methoxy-3-(octadecyloxy)- involves its interaction with molecular targets such as enzymes, receptors, or other biomolecules. The specific pathways and molecular targets depend on the context of its application. For example, in biological systems, it may interact with cell membranes or proteins, affecting their function and activity .
Comparaison Avec Des Composés Similaires
Structural Analogues: Ether-Linked Glycerols
1-O-Octadecyl-rac-glycerol (Batyl Alcohol)
- Structure : Lacks the 2-O-methyl group, featuring a hydroxyl group at sn-2.
- Activity: Less cytotoxic than the methylated analogue due to faster metabolic clearance.
- Metabolism : Susceptible to phospholipase-mediated hydrolysis, reducing its intracellular retention .
1-O-Hexadecyl-2-O-methyl-rac-glycerol
- Structure : Shorter alkyl chain (C16 vs. C18) with a 2-O-methyl group.
- Activity : Reduced membrane integration and cytotoxicity compared to the C18 analogue, highlighting the importance of alkyl chain length for lipid solubility and target binding .
Phosphocholine Derivatives
1-Octadecyl-2-methyl-rac-glycero-3-phosphocholine (ET-18-OCH3)
- Structure : Contains a phosphocholine head group at sn-3.
- Activity: Potent inhibitor of inositol phosphate-mediated Ca²⁺ signaling (IC₅₀ = 19 µM for cell growth inhibition). The phosphocholine group enhances membrane interaction but increases metabolic susceptibility .
- Metabolism : Undergoes phospholipase C/D-mediated cleavage, generating cytotoxic lysophospholipids .
rac-1-Hexadecyl-2-methoxy-glycero-3-phosphocholine
- Structure : C16 alkyl chain with 2-methoxy and phosphocholine groups.
- Activity : Similar mechanism to ET-18-OCH3 but with lower potency due to shorter alkyl chain .
Ester-Based Glycerides
1-Stearoyl-rac-glycerol
- Structure : Ester-linked stearic acid (C18:0) at sn-1.
- Activity : Lacks antitumor activity due to rapid hydrolysis by lipases. Demonstrates the critical role of ether linkages in metabolic stability .
1,3-Dioleoyl-2-palmitoylglycerol
- Structure : Triacylglycerol with ester bonds at all positions.
Metabolic Stability and Selectivity
- 1-O-Octadecyl-2-O-methyl-rac-glycerol : Minimal metabolism in tumor cells (<2% degradation in 24 hours) due to resistance to alkyl cleavage enzymes and phospholipases. This results in selective accumulation and cytotoxicity .
- Phosphocholine Analogues (e.g., ET-18-OCH3): Extensive metabolism in normal tissues (e.g., liver), generating stearyl alcohol and other metabolites, which limits in vivo efficacy .
- Key Enzymatic Differences :
- Alkyl cleavage enzyme activity is similar across cell types, indicating that cytotoxicity differences arise from uptake and retention rather than enzymatic degradation .
- The 2-O-methyl group in the target compound prevents reacylation by lysophosphatidylcholine acyltransferase, further promoting lysophospholipid accumulation .
Cytotoxicity and Selectivity Data
Mechanism of Action Comparison
Activité Biologique
1-O-Octadecyl-2-O-methyl-rac-glycerol (OMG) is a synthetic ether lipid that has garnered attention for its potential biological activities, particularly in the context of cancer therapy. This compound is structurally related to other alkyl-lysophospholipids and exhibits a range of effects on cellular processes, including apoptosis and signal transduction pathways. This article provides a comprehensive overview of OMG's biological activity, supported by research findings, data tables, and case studies.
OMG has been studied primarily for its antitumor properties. It is known to selectively induce apoptosis in tumor cells while sparing normal cells, a characteristic that makes it a candidate for cancer treatment. The mechanism of action appears to involve the incorporation of OMG into cellular membranes, leading to the activation of apoptotic pathways.
The biological activity of OMG can be attributed to several key mechanisms:
- Cell Membrane Interaction : OMG integrates into the lipid bilayer of cell membranes, altering membrane dynamics and function.
- Apoptosis Induction : The compound activates the Fas/CD95 receptor pathway, which is crucial for apoptosis in tumor cells. This selective targeting is significant as normal cells show limited uptake of OMG, thus avoiding unwanted cytotoxic effects .
- MAPK Pathway Inhibition : OMG inhibits the mitogen-activated protein kinase (MAPK) signaling cascade, which is often overactive in cancer cells. This inhibition contributes to its ability to induce apoptosis selectively in malignant cells .
Case Studies
- Tumor Cell Lines : In studies involving human leukemia cell lines (U937 and Jurkat T), OMG was shown to induce apoptosis in a dose-dependent manner. The combination of OMG with ionizing radiation further enhanced apoptotic effects, suggesting a potential strategy for improving cancer therapies through combination treatments .
- Normal vs. Tumor Cells : Research indicates that normal cells, such as human fibroblasts, do not significantly incorporate OMG and are resistant to its apoptotic effects unless the compound is directly microinjected into them. This highlights the selective nature of OMG’s action against tumor cells .
Data Table: Biological Activity Overview
Additional Biological Activities
Beyond its antitumor effects, OMG has been explored for its roles in other biomedical contexts:
- Antiparasitic Activity : Some studies suggest that ether lipids like OMG may have therapeutic potential against parasitic infections due to their ability to disrupt membrane integrity in target organisms .
- Autoimmune Diseases : Research indicates that these compounds may also modulate immune responses, providing avenues for treatment in autoimmune conditions .
Q & A
Basic Research Questions
Q. What are the optimal synthetic routes for 1-O-Octadecyl-2-O-methyl-rac-glycerol, and how can regioselectivity be ensured during synthesis?
- Methodological Answer : The synthesis typically involves regioselective protection of glycerol hydroxyl groups. For example, acetonide or benzylidene protecting groups can stabilize the 1,2- or 1,3-positions of glycerol, allowing selective alkylation or acylation at the desired position . Ether linkages (e.g., octadecyl groups) are introduced via nucleophilic substitution using alkyl halides or Mitsunobu reactions, while methyl groups at the 2-position require mild methylation agents like methyl iodide under basic conditions. Yield optimization often depends on reaction temperature (e.g., 0–25°C) and catalysts (e.g., BF₃·Et₂O for ether formation) .
Q. What analytical techniques are most effective for characterizing this compound?
- Methodological Answer :
- Thin-Layer Chromatography (TLC) : Use silica gel plates with a mobile phase of chloroform:methanol (9:1) to monitor reaction progress .
- NMR Spectroscopy : ¹H and ¹³C NMR are critical for confirming regiochemistry. For example, the methyl group at the 2-position shows a distinct singlet at ~3.3 ppm, while the octadecyl chain’s terminal methyl resonates at 0.8–1.0 ppm .
- Mass Spectrometry (MS) : High-resolution ESI-MS or MALDI-TOF can verify molecular weight (expected m/z: ~400–450) and detect impurities .
Q. What safety protocols are essential when handling this compound in the laboratory?
- Methodological Answer :
- Personal Protective Equipment (PPE) : Wear nitrile gloves, lab coats, and safety goggles to avoid skin/eye contact. Use explosion-proof equipment if volatile solvents are involved .
- Ventilation : Conduct reactions in a fume hood to minimize inhalation risks, especially during alkylation steps .
- Waste Disposal : Collect organic waste in designated containers for incineration, adhering to local regulations .
Advanced Research Questions
Q. How does the ether linkage in this compound influence its monolayer formation and membrane interactions compared to ester analogs?
- Methodological Answer : Ether linkages enhance chemical stability and reduce hydrolysis. Use Langmuir-Blodgett troughs to compare monolayer properties (e.g., surface pressure-area isotherms) with ester analogs. Ether-containing lipids typically exhibit higher collapse pressures (~45–50 mN/m) due to stronger van der Waals interactions between alkyl chains . For bilayer studies, employ differential scanning calorimetry (DSC) to analyze phase transition temperatures, which are often 5–10°C higher for ether-linked lipids .
Q. How can stereochemical purity (rac vs. sn-configurations) impact the biological activity of 1-O-Octadecyl-2-O-methyl-glycerol derivatives?
- Methodological Answer : Stereochemistry affects receptor binding and metabolic pathways. To assess this:
- Enzymatic Assays : Test sn-1 vs. rac isomers against phospholipases (e.g., PLA₂). Rac mixtures may show reduced activity due to steric hindrance .
- Cell Culture Models : Compare membrane incorporation efficiency in lipid rafts using fluorescently tagged analogs. sn-configurations often align better with natural phospholipid packing .
Q. What experimental strategies resolve discrepancies in NMR or MS data when synthesizing this compound?
- Methodological Answer :
- Contamination Check : Run a blank NMR/MS to rule out solvent or column bleed artifacts .
- Isotopic Labeling : Synthesize deuterated analogs (e.g., CD₃-O- groups) to distinguish overlapping peaks in complex spectra .
- 2D NMR : Use HSQC or COSY to resolve signal overlaps, particularly for glycerol backbone protons .
Propriétés
IUPAC Name |
2-methoxy-3-octadecoxypropan-1-ol | |
---|---|---|
Source | PubChem | |
URL | https://pubchem.ncbi.nlm.nih.gov | |
Description | Data deposited in or computed by PubChem | |
InChI |
InChI=1S/C22H46O3/c1-3-4-5-6-7-8-9-10-11-12-13-14-15-16-17-18-19-25-21-22(20-23)24-2/h22-23H,3-21H2,1-2H3 | |
Source | PubChem | |
URL | https://pubchem.ncbi.nlm.nih.gov | |
Description | Data deposited in or computed by PubChem | |
InChI Key |
JWBOVDKFGDXGCR-UHFFFAOYSA-N | |
Source | PubChem | |
URL | https://pubchem.ncbi.nlm.nih.gov | |
Description | Data deposited in or computed by PubChem | |
Canonical SMILES |
CCCCCCCCCCCCCCCCCCOCC(CO)OC | |
Source | PubChem | |
URL | https://pubchem.ncbi.nlm.nih.gov | |
Description | Data deposited in or computed by PubChem | |
Molecular Formula |
C22H46O3 | |
Source | PubChem | |
URL | https://pubchem.ncbi.nlm.nih.gov | |
Description | Data deposited in or computed by PubChem | |
DSSTOX Substance ID |
DTXSID70319792 | |
Record name | 1-Propanol, 2-methoxy-3-(octadecyloxy)- | |
Source | EPA DSSTox | |
URL | https://comptox.epa.gov/dashboard/DTXSID70319792 | |
Description | DSSTox provides a high quality public chemistry resource for supporting improved predictive toxicology. | |
Molecular Weight |
358.6 g/mol | |
Source | PubChem | |
URL | https://pubchem.ncbi.nlm.nih.gov | |
Description | Data deposited in or computed by PubChem | |
CAS No. |
84337-43-9 | |
Record name | NSC350624 | |
Source | DTP/NCI | |
URL | https://dtp.cancer.gov/dtpstandard/servlet/dwindex?searchtype=NSC&outputformat=html&searchlist=350624 | |
Description | The NCI Development Therapeutics Program (DTP) provides services and resources to the academic and private-sector research communities worldwide to facilitate the discovery and development of new cancer therapeutic agents. | |
Explanation | Unless otherwise indicated, all text within NCI products is free of copyright and may be reused without our permission. Credit the National Cancer Institute as the source. | |
Record name | 1-Propanol, 2-methoxy-3-(octadecyloxy)- | |
Source | EPA DSSTox | |
URL | https://comptox.epa.gov/dashboard/DTXSID70319792 | |
Description | DSSTox provides a high quality public chemistry resource for supporting improved predictive toxicology. | |
Avertissement et informations sur les produits de recherche in vitro
Veuillez noter que tous les articles et informations sur les produits présentés sur BenchChem sont destinés uniquement à des fins informatives. Les produits disponibles à l'achat sur BenchChem sont spécifiquement conçus pour des études in vitro, qui sont réalisées en dehors des organismes vivants. Les études in vitro, dérivées du terme latin "in verre", impliquent des expériences réalisées dans des environnements de laboratoire contrôlés à l'aide de cellules ou de tissus. Il est important de noter que ces produits ne sont pas classés comme médicaments et n'ont pas reçu l'approbation de la FDA pour la prévention, le traitement ou la guérison de toute condition médicale, affection ou maladie. Nous devons souligner que toute forme d'introduction corporelle de ces produits chez les humains ou les animaux est strictement interdite par la loi. Il est essentiel de respecter ces directives pour assurer la conformité aux normes légales et éthiques en matière de recherche et d'expérimentation.