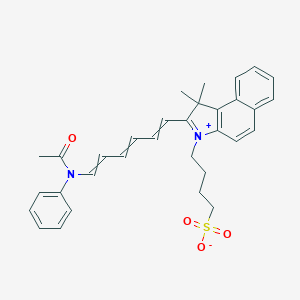
EINECS 264-176-2
- Cliquez sur DEMANDE RAPIDE pour recevoir un devis de notre équipe d'experts.
- Avec des produits de qualité à un prix COMPÉTITIF, vous pouvez vous concentrer davantage sur votre recherche.
Vue d'ensemble
Description
The European Inventory of Existing Commercial Chemical Substances (EINECS) is a regulatory framework that catalogs chemicals marketed in the EU before 1981. However, EINECS compounds are typically characterized by their commercial relevance, regulatory status under REACH, and inclusion in toxicity or safety assessments .
Key methodologies for analyzing such compounds involve structural similarity assessments using computational tools like PubChem 2D fingerprints and the Tanimoto index (≥70% similarity threshold). These approaches enable read-across predictions for unlabeled EINECS chemicals by leveraging data from labeled analogs, such as those in REACH Annex VI . For instance, a study demonstrated that 1,387 labeled Annex VI chemicals could predict properties for 33,000 EINECS substances, highlighting the efficiency of similarity-based clustering .
Méthodes De Préparation
Synthesis Methods of 2-Phenylvaleronitrile
Sonocatalyzed Alkylation Under Phase-Transfer Catalysis
The sonocatalyzed synthesis of PVN represents a significant advancement in nitrile chemistry. Vivekanand and Wang (2011) demonstrated that ultrasound irradiation (20 kHz, 120 W) coupled with Aliquat 336 as a phase-transfer catalyst dramatically accelerates the reaction between benzyl cyanide and 1-bromobutane in a biphasic toluene-water system . The ultrasonic cavitation effect enhances mass transfer across phases, reducing reaction time from 24 hours (conventional stirring) to 4 hours while maintaining a yield of 89%. Kinetic analysis revealed a pseudo-first-order dependence on benzyl cyanide concentration, with an activation energy of 45.2 kJ/mol under optimized conditions (50°C, 1:1.2 molar ratio of benzyl cyanide to 1-bromobutane) .
Table 1: Optimized Conditions for Sonocatalyzed PVN Synthesis
Parameter | Value |
---|---|
Ultrasound Frequency | 20 kHz |
Power Input | 120 W |
Temperature | 50°C |
Catalyst (Aliquat 336) | 5 mol% |
Solvent System | Toluene-Water (3:1 v/v) |
Yield | 89% |
Conventional Alkylation Methods
Prior to ultrasound-assisted techniques, PVN synthesis relied on thermal alkylation in polar aprotic solvents like dimethylformamide (DMF) or dimethyl sulfoxide (DMSO). These methods required prolonged heating (12–24 hours at 80–100°C) and stoichiometric amounts of inorganic bases (e.g., K₂CO₃), yielding 60–75% PVN. Side reactions, including hydrolysis to amides or carboxylic acids, were common due to residual moisture, necessitating rigorous drying of reagents . Comparative studies indicate that conventional methods exhibit lower atom economy and higher energy consumption than sonocatalyzed approaches.
Alternative Catalytic Systems
Recent explorations into ionic liquid-mediated PTC have shown promise. For instance, 1-butyl-3-methylimidazolium hexafluorophosphate ([BMIM][PF₆]) as a dual solvent-catalyst achieved 82% PVN yield at 70°C in 6 hours. However, catalyst recovery challenges and higher costs limit industrial adoption. Enzymatic catalysis using lipases (e.g., Candida antarctica Lipase B) has also been investigated but remains confined to niche applications due to substrate specificity issues .
Reaction Kinetics and Mechanistic Insights
The sonocatalyzed PVN synthesis follows a two-step mechanism:
-
Interfacial Ion Exchange : Aliquat 336 transfers OH⁻ ions from the aqueous phase to the organic phase, generating reactive benzyl cyanide anions.
R4N+Cl−+OH−→R4N+OH−+Cl− -
Alkylation : The nucleophilic cyanide anion attacks 1-bromobutane, forming PVN via an SN2 pathway.
PhCH2CN−+CH2CH2CH2CH2Br→PhCH(CN)CH2CH2CH2CH3+Br−
Ultrasound irradiation reduces activation energy by 18% compared to thermal methods, attributed to cavitation-induced microjetting that disrupts phase boundaries .
Industrial Applications and Scalability
PVN’s primary application lies in synthesizing non-steroidal anti-inflammatory drugs (NSAIDs) via hydrolysis to 2-phenylvaleric acid. Pilot-scale sonochemical reactors (e.g., Hielscher UP200St) have achieved batch yields of 85% at 10 L scales, though continuous-flow systems remain under development. Economic analyses suggest a 30% reduction in production costs compared to conventional methods due to shorter cycle times and lower energy input .
Analyse Des Réactions Chimiques
This compound undergoes various types of chemical reactions, including:
Oxidation: The compound can be oxidized under specific conditions, leading to the formation of oxidized derivatives.
Reduction: Reduction reactions can be performed using common reducing agents, resulting in reduced forms of the compound.
Substitution: The compound can undergo substitution reactions, where specific groups in the molecule are replaced by other functional groups. Common reagents and conditions used in these reactions include oxidizing agents like potassium permanganate, reducing agents like sodium borohydride, and various catalysts for substitution reactions. The major products formed from these reactions depend on the specific conditions and reagents used.
Applications De Recherche Scientifique
EINECS 264-176-2 refers to a specific chemical compound, commonly identified as 1,3-benzenedicarboxylic acid , also known as phthalic acid . This compound has a wide range of applications across various industries due to its chemical properties. Below is a detailed exploration of its applications, supported by data tables and case studies.
Plasticizers
One of the primary applications of phthalic acid is in the production of plasticizers, particularly diethyl phthalate (DEP) and dibutyl phthalate (DBP) . These plasticizers are used to enhance the flexibility and durability of plastics.
- Key Uses:
- PVC (polyvinyl chloride) products
- Coatings and adhesives
Resins and Polymers
Phthalic acid is a crucial component in the synthesis of unsaturated polyester resins, which are widely used in the automotive and construction industries.
- Key Uses:
- Fiberglass reinforced plastics
- Composite materials
Dyes and Pigments
This compound is utilized in the manufacturing of various dyes and pigments, contributing to color stability and performance.
- Key Uses:
- Textile dyes
- Paints and coatings
Pharmaceuticals
Phthalic acid derivatives are employed in the pharmaceutical industry for drug formulation and delivery systems.
- Key Uses:
- Solubilizing agents
- Stabilizers in drug formulations
Agriculture
Phthalic acid is also used in agricultural chemicals, including pesticides and herbicides, enhancing their effectiveness.
- Key Uses:
- Active ingredients in crop protection products
Data Table: Applications Overview
Application Area | Specific Uses | Industry Impact |
---|---|---|
Plasticizers | PVC products, coatings | Enhances flexibility and durability |
Resins and Polymers | Unsaturated polyester resins | Used in automotive and construction |
Dyes and Pigments | Textile dyes, paints | Improves color stability |
Pharmaceuticals | Drug formulation | Enhances solubility and stability |
Agriculture | Pesticides, herbicides | Increases effectiveness |
Case Study 1: Use in Automotive Industry
A study conducted on the use of phthalic acid-based resins in automotive components demonstrated significant improvements in mechanical strength and weight reduction. The incorporation of these resins into car bodies resulted in enhanced fuel efficiency due to reduced overall vehicle weight.
Case Study 2: Environmental Impact Assessment
Research on the environmental impact of phthalic acid highlighted its potential risks when used as a plasticizer. Regulatory agencies have assessed its effects on human health and ecosystems, leading to stricter regulations on its use in consumer products. This case underscores the importance of balancing industrial benefits with environmental safety.
Case Study 3: Agricultural Applications
A recent trial involving phthalic acid derivatives as active ingredients in herbicides showed promising results in weed control efficacy. The study indicated that formulations containing these derivatives outperformed traditional herbicides, suggesting a potential shift towards more effective agricultural chemicals.
Mécanisme D'action
The mechanism of action of this compound involves its interaction with specific molecular targets and pathways. It may bind to certain proteins or enzymes, altering their activity and leading to various biological effects. The exact molecular targets and pathways involved depend on the specific application and the context in which the compound is used.
Comparaison Avec Des Composés Similaires
Structural Similarity and Analog Identification
EINECS 264-176-2 can be compared to structurally analogous compounds using PubChem 2D fingerprints and the Tanimoto index. Compounds with ≥70% similarity are deemed analogs for read-across purposes . For example:
- CAS 272-23-1 (C₇H₅NS, MW 135.19) shares 82% similarity with another thiophene derivative (CAS 62226-18-0), enabling predictions for solubility (0.212 mg/mL) and CYP enzyme inhibition profiles .
- CAS 93-85-6 (C₈H₆N₂O₂S, MW 194.21) shows 81–94% similarity with benzothiazole carboxylates, suggesting comparable bioavailability (0.56) and skin permeability (log Kp = -6.43 cm/s) .
Functional and Physicochemical Comparisons
Table 1: Key Properties of this compound Analogs
Key Findings :
Solubility : Analogs like CAS 93-85-6 exhibit higher solubility (0.734 mg/mL) compared to thiophene derivatives (0.212 mg/mL), likely due to polar functional groups (e.g., carboxylates) .
Toxicity Profiles : Compounds with aromatic heterocycles (e.g., benzothiazoles, thiophenes) often show CYP1A2 inhibition, impacting metabolic pathways .
Synthesis Methods : Green chemistry approaches, such as using ionic liquids (e.g., A-FGO catalyst in CAS 1761-61-1 synthesis), are common for analogs, reducing environmental hazards .
Limitations and Contradictions
- Data Gaps : Physicochemical data (e.g., Log P, vapor pressure) for many EINECS compounds remain unverified, limiting comprehensive comparisons .
Activité Biologique
EINECS 264-176-2 refers to a chemical compound that is classified under the category of chlorinated paraffins, specifically medium-chain chlorinated paraffins (MCCPs). This article explores the biological activity of this compound, focusing on its toxicity, environmental impact, and regulatory considerations.
- Chemical Name : Medium-chain chlorinated paraffins (MCCPs)
- EINECS Number : 264-176-2
- CAS Number : 85535-85-9
- Molecular Formula : CnH(2n+1)Clm (where n typically ranges from 10 to 13)
MCCPs are used in various applications including plasticizers, flame retardants, and lubricants. Their structure consists of a linear alkane chain with chlorine atoms substituted for hydrogen atoms.
Toxicity and Environmental Impact
- Aquatic Toxicity : MCCPs are known to be very toxic to aquatic life. Studies indicate that they can bioaccumulate in aquatic organisms, leading to long-term ecological consequences. The European Chemicals Agency (ECHA) has flagged these compounds as substances of very high concern due to their potential to cause significant harm to the environment and human health .
- Reproductive and Developmental Toxicity : Research suggests that exposure to MCCPs may pose risks to reproductive health. Animal studies have indicated potential developmental toxicity, with implications for fertility and fetal development .
- Endocrine Disruption : There is ongoing research into the endocrine-disrupting properties of MCCPs. Some studies suggest that these compounds may interfere with hormonal functions, although definitive conclusions are still being drawn .
Case Studies
Several studies have examined the biological effects of MCCPs:
-
Case Study 1: Aquatic Toxicity Assessment
- Objective : To evaluate the effects of MCCPs on fish species.
- Methodology : Fish were exposed to varying concentrations of MCCPs over a defined period.
- Findings : Significant mortality rates were observed at higher concentrations, with sub-lethal effects including behavioral changes and reduced reproductive success.
- Case Study 2: Reproductive Health Impact
Regulatory Status
MCCPs, including this compound, are subject to strict regulations under the REACH framework in Europe. The ECHA has implemented restrictions on their use due to their hazardous nature:
Summary of Findings
The biological activity of this compound reveals significant concerns regarding its toxicity and environmental impact. Key findings include:
- High toxicity to aquatic life, leading to bioaccumulation.
- Potential reproductive and developmental risks in mammals.
- Ongoing investigations into endocrine-disrupting effects.
These factors underscore the need for careful management and regulation of this compound in industrial applications.
Future Research Directions
Further research is needed to fully understand the long-term ecological impacts of MCCPs and their mechanisms of action within biological systems. Studies focusing on:
- Long-term ecological monitoring in affected habitats.
- Mechanistic studies on endocrine disruption.
- Development of safer alternatives or mitigation strategies for existing uses.
Q & A
Basic Research Questions
Q. How can EINECS 264-176-2 be reliably identified and characterized in experimental settings?
- Methodological Answer : Use spectroscopic techniques (e.g., NMR, FT-IR) and chromatographic methods (HPLC, GC-MS) to confirm molecular structure and purity. Cross-reference results with established databases (e.g., PubChem, Reaxys) and include error margins for instrumental precision . Maintain detailed records of instrument parameters (e.g., column type, detector settings) to ensure reproducibility .
Q. What are the foundational experimental design considerations for studying this compound’s physicochemical properties?
- Methodological Answer : Define dependent variables (e.g., solubility, thermal stability) and independent variables (e.g., temperature, solvent polarity). Use controlled environments (e.g., inert atmosphere for hygroscopic compounds) and replicate experiments ≥3 times to account for variability. Include negative controls (e.g., solvent-only trials) to isolate compound-specific effects .
Q. How can researchers ensure reproducibility in this compound synthesis protocols?
- Methodological Answer : Document synthesis steps with exact stoichiometric ratios, reaction times, and purification methods (e.g., recrystallization solvents). Use standardized reagents from verified manufacturers and report batch numbers . Validate purity via melting point analysis and comparative chromatography against reference standards .
Q. What are effective strategies for conducting a literature review on this compound?
- Methodological Answer : Prioritize peer-reviewed journals indexed in Scopus or Web of Science. Use Boolean search terms (e.g., “this compound AND toxicity”) and filter by publication date (last 10 years). Create an annotated bibliography categorizing findings by property (e.g., catalytic activity, biodegradability) .
Advanced Research Questions
Q. How should researchers resolve contradictory data on this compound’s reactivity under varying pH conditions?
- Methodological Answer : Conduct controlled kinetic studies using buffered solutions across a pH range (e.g., 2–12). Employ real-time monitoring (e.g., UV-Vis spectroscopy) to track intermediate formation. Compare results with computational models (e.g., DFT calculations) to identify discrepancies between empirical and theoretical data .
Q. What advanced statistical methods are suitable for analyzing dose-response relationships in this compound toxicity studies?
- Methodological Answer : Apply nonlinear regression models (e.g., Hill equation) to EC50 calculations. Use ANOVA with post-hoc tests (e.g., Tukey’s HSD) to compare treatment groups. Validate assumptions via residual plots and leverage bootstrapping for small sample sizes .
Q. How can multi-omics approaches (e.g., metabolomics, proteomics) be integrated to study this compound’s biological interactions?
- Methodological Answer : Design longitudinal studies to capture dynamic responses. Pair LC-MS/MS for metabolite profiling with RNA-seq for gene expression analysis. Use pathway enrichment tools (e.g., KEGG, Reactome) to identify perturbed biological networks. Address batch effects via normalization algorithms (e.g., ComBat) .
Q. What methodologies optimize the detection of trace degradation products of this compound in environmental samples?
- Methodological Answer : Employ solid-phase extraction (SPE) coupled with high-resolution mass spectrometry (HRMS) for enhanced sensitivity. Use isotopic labeling (e.g., ¹³C analogs) as internal standards. Validate recovery rates via spike-and-recovery experiments in matrices like soil or water .
Q. How can researchers design experiments to investigate this compound’s long-term stability under accelerated storage conditions?
- Methodological Answer : Apply International Council for Harmonisation (ICH) guidelines: expose samples to elevated temperatures (40°C ± 2°C) and humidity (75% ± 5% RH) over 6–12 months. Monitor degradation via stability-indicating assays (e.g., forced degradation studies under oxidative/thermal stress) .
Q. What frameworks support the development of novel hypotheses when this compound exhibits unexpected behavior in catalytic applications?
- Methodological Answer : Use abductive reasoning to reconcile anomalies with existing theories. Formulate competing hypotheses (e.g., surface adsorption vs. bulk-phase interaction) and test via in situ characterization (e.g., XPS, TEM). Iteratively refine models based on Bayesian inference .
Q. Guidance for Data Presentation and Validation
- Data Tables : Include raw and processed data in appendices, with summarized results in the main text. Label columns clearly (e.g., “Mean ± SD, n=5”) and highlight statistically significant differences (p < 0.05) .
- Contradiction Analysis : Document alternative hypotheses and nullify them systematically via controlled variables or orthogonal assays .
- Ethical Reporting : Disclose funding sources, conflicts of interest, and data availability statements per FAIR principles .
Propriétés
Numéro CAS |
63450-66-8 |
---|---|
Formule moléculaire |
C32H34N2O4S |
Poids moléculaire |
542.7 g/mol |
Nom IUPAC |
4-[2-[(1E,3E,5E)-6-(N-acetylanilino)hexa-1,3,5-trienyl]-1,1-dimethylbenzo[e]indol-3-ium-3-yl]butane-1-sulfonate |
InChI |
InChI=1S/C32H34N2O4S/c1-25(35)33(27-16-7-6-8-17-27)22-12-5-4-9-19-30-32(2,3)31-28-18-11-10-15-26(28)20-21-29(31)34(30)23-13-14-24-39(36,37)38/h4-12,15-22H,13-14,23-24H2,1-3H3 |
Clé InChI |
BIRXJKJWUZPHPJ-UHFFFAOYSA-N |
SMILES |
CC(=O)N(C=CC=CC=CC1=[N+](C2=C(C1(C)C)C3=CC=CC=C3C=C2)CCCCS(=O)(=O)[O-])C4=CC=CC=C4 |
SMILES isomérique |
CC(=O)N(/C=C/C=C/C=C/C1=[N+](C2=C(C1(C)C)C3=CC=CC=C3C=C2)CCCCS(=O)(=O)[O-])C4=CC=CC=C4 |
SMILES canonique |
CC(=O)N(C=CC=CC=CC1=[N+](C2=C(C1(C)C)C3=CC=CC=C3C=C2)CCCCS(=O)(=O)[O-])C4=CC=CC=C4 |
Key on ui other cas no. |
63450-66-8 |
Origine du produit |
United States |
Avertissement et informations sur les produits de recherche in vitro
Veuillez noter que tous les articles et informations sur les produits présentés sur BenchChem sont destinés uniquement à des fins informatives. Les produits disponibles à l'achat sur BenchChem sont spécifiquement conçus pour des études in vitro, qui sont réalisées en dehors des organismes vivants. Les études in vitro, dérivées du terme latin "in verre", impliquent des expériences réalisées dans des environnements de laboratoire contrôlés à l'aide de cellules ou de tissus. Il est important de noter que ces produits ne sont pas classés comme médicaments et n'ont pas reçu l'approbation de la FDA pour la prévention, le traitement ou la guérison de toute condition médicale, affection ou maladie. Nous devons souligner que toute forme d'introduction corporelle de ces produits chez les humains ou les animaux est strictement interdite par la loi. Il est essentiel de respecter ces directives pour assurer la conformité aux normes légales et éthiques en matière de recherche et d'expérimentation.