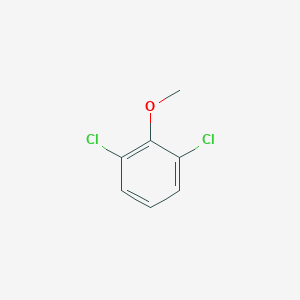
2,6-Dichloroanisole
Vue d'ensemble
Description
GR218231 est un composé organique synthétique connu pour son rôle d’antagoniste du récepteur de la dopamine D3Le composé a montré un potentiel prometteur pour visualiser et quantifier l’activité de la P-glycoprotéine dans la barrière hémato-encéphalique .
Applications De Recherche Scientifique
GR218231 has been utilized in various scientific research applications:
Mécanisme D'action
GR218231 exerce ses effets en antagonisant les récepteurs de la dopamine D3. Cette interaction inhibe l’activité du récepteur, ce qui peut être utile pour étudier diverses affections neurologiques. De plus, l’interaction du composé avec la P-glycoprotéine suggère qu’il peut influencer l’efflux des médicaments et d’autres substances à travers la barrière hémato-encéphalique .
Méthodes De Préparation
La synthèse du GR218231 implique la réaction d’un précurseur déméthylé avec du triflate de 11C-méthyle. Cette méthode a été utilisée pour marquer le composé avec du carbone-11, un isotope émetteur de positrons, le rendant adapté à l’imagerie TEP . La préparation produit un produit radiochimique ayant une activité spécifique de 15 ± 10 GBq/µmol .
Analyse Des Réactions Chimiques
GR218231 subit plusieurs types de réactions chimiques, notamment :
Réactions de substitution : Le composé peut être marqué avec du carbone-11 par une réaction de substitution impliquant du triflate de 11C-méthyle.
Réactions de liaison : Il se lie spécifiquement aux récepteurs de la dopamine D3, bien qu’il ait également été démontré qu’il interagissait avec la P-glycoprotéine.
Applications de recherche scientifique
GR218231 a été utilisé dans diverses applications de recherche scientifique :
Neurosciences : Il a été utilisé comme traceur TEP pour étudier la densité et l’occupation des récepteurs de la dopamine D3.
Développement de médicaments : Sa capacité à visualiser l’activité de la P-glycoprotéine en fait un outil précieux dans le développement de nouveaux médicaments et de stratégies pour surmonter la résistance aux médicaments.
Comparaison Avec Des Composés Similaires
GR218231 est unique dans son double rôle d’antagoniste du récepteur de la dopamine D3 et de substrat pour la P-glycoprotéine. Les composés similaires comprennent :
Bifeprunox : Un agoniste partiel des récepteurs de la dopamine D2 et D3.
Aripiprazole : Un autre agoniste partiel des récepteurs de la dopamine D2 et D3.
Ces composés partagent certaines propriétés pharmacologiques avec GR218231, mais diffèrent dans leurs interactions spécifiques avec les récepteurs et leurs applications.
Propriétés
IUPAC Name |
1,3-dichloro-2-methoxybenzene | |
---|---|---|
Source | PubChem | |
URL | https://pubchem.ncbi.nlm.nih.gov | |
Description | Data deposited in or computed by PubChem | |
InChI |
InChI=1S/C7H6Cl2O/c1-10-7-5(8)3-2-4-6(7)9/h2-4H,1H3 | |
Source | PubChem | |
URL | https://pubchem.ncbi.nlm.nih.gov | |
Description | Data deposited in or computed by PubChem | |
InChI Key |
KZLMCDNAVVJKPX-UHFFFAOYSA-N | |
Source | PubChem | |
URL | https://pubchem.ncbi.nlm.nih.gov | |
Description | Data deposited in or computed by PubChem | |
Canonical SMILES |
COC1=C(C=CC=C1Cl)Cl | |
Source | PubChem | |
URL | https://pubchem.ncbi.nlm.nih.gov | |
Description | Data deposited in or computed by PubChem | |
Molecular Formula |
C7H6Cl2O | |
Source | PubChem | |
URL | https://pubchem.ncbi.nlm.nih.gov | |
Description | Data deposited in or computed by PubChem | |
DSSTOX Substance ID |
DTXSID1062097 | |
Record name | 2,6-Dichloroanisole | |
Source | EPA DSSTox | |
URL | https://comptox.epa.gov/dashboard/DTXSID1062097 | |
Description | DSSTox provides a high quality public chemistry resource for supporting improved predictive toxicology. | |
Molecular Weight |
177.02 g/mol | |
Source | PubChem | |
URL | https://pubchem.ncbi.nlm.nih.gov | |
Description | Data deposited in or computed by PubChem | |
CAS No. |
1984-65-2 | |
Record name | 2,6-Dichloroanisole | |
Source | CAS Common Chemistry | |
URL | https://commonchemistry.cas.org/detail?cas_rn=1984-65-2 | |
Description | CAS Common Chemistry is an open community resource for accessing chemical information. Nearly 500,000 chemical substances from CAS REGISTRY cover areas of community interest, including common and frequently regulated chemicals, and those relevant to high school and undergraduate chemistry classes. This chemical information, curated by our expert scientists, is provided in alignment with our mission as a division of the American Chemical Society. | |
Explanation | The data from CAS Common Chemistry is provided under a CC-BY-NC 4.0 license, unless otherwise stated. | |
Record name | Benzene, 1,3-dichloro-2-methoxy- | |
Source | ChemIDplus | |
URL | https://pubchem.ncbi.nlm.nih.gov/substance/?source=chemidplus&sourceid=0001984652 | |
Description | ChemIDplus is a free, web search system that provides access to the structure and nomenclature authority files used for the identification of chemical substances cited in National Library of Medicine (NLM) databases, including the TOXNET system. | |
Record name | Benzene, 1,3-dichloro-2-methoxy- | |
Source | EPA Chemicals under the TSCA | |
URL | https://www.epa.gov/chemicals-under-tsca | |
Description | EPA Chemicals under the Toxic Substances Control Act (TSCA) collection contains information on chemicals and their regulations under TSCA, including non-confidential content from the TSCA Chemical Substance Inventory and Chemical Data Reporting. | |
Record name | 2,6-Dichloroanisole | |
Source | EPA DSSTox | |
URL | https://comptox.epa.gov/dashboard/DTXSID1062097 | |
Description | DSSTox provides a high quality public chemistry resource for supporting improved predictive toxicology. | |
Record name | 2,6-dichloroanisole | |
Source | European Chemicals Agency (ECHA) | |
URL | https://echa.europa.eu/substance-information/-/substanceinfo/100.016.233 | |
Description | The European Chemicals Agency (ECHA) is an agency of the European Union which is the driving force among regulatory authorities in implementing the EU's groundbreaking chemicals legislation for the benefit of human health and the environment as well as for innovation and competitiveness. | |
Explanation | Use of the information, documents and data from the ECHA website is subject to the terms and conditions of this Legal Notice, and subject to other binding limitations provided for under applicable law, the information, documents and data made available on the ECHA website may be reproduced, distributed and/or used, totally or in part, for non-commercial purposes provided that ECHA is acknowledged as the source: "Source: European Chemicals Agency, http://echa.europa.eu/". Such acknowledgement must be included in each copy of the material. ECHA permits and encourages organisations and individuals to create links to the ECHA website under the following cumulative conditions: Links can only be made to webpages that provide a link to the Legal Notice page. | |
Retrosynthesis Analysis
AI-Powered Synthesis Planning: Our tool employs the Template_relevance Pistachio, Template_relevance Bkms_metabolic, Template_relevance Pistachio_ringbreaker, Template_relevance Reaxys, Template_relevance Reaxys_biocatalysis model, leveraging a vast database of chemical reactions to predict feasible synthetic routes.
One-Step Synthesis Focus: Specifically designed for one-step synthesis, it provides concise and direct routes for your target compounds, streamlining the synthesis process.
Accurate Predictions: Utilizing the extensive PISTACHIO, BKMS_METABOLIC, PISTACHIO_RINGBREAKER, REAXYS, REAXYS_BIOCATALYSIS database, our tool offers high-accuracy predictions, reflecting the latest in chemical research and data.
Strategy Settings
Precursor scoring | Relevance Heuristic |
---|---|
Min. plausibility | 0.01 |
Model | Template_relevance |
Template Set | Pistachio/Bkms_metabolic/Pistachio_ringbreaker/Reaxys/Reaxys_biocatalysis |
Top-N result to add to graph | 6 |
Feasible Synthetic Routes
Q1: What is the molecular structure and spectroscopic data available for 2,6-Dichloroanisole?
A1: this compound (DCA) has the molecular formula C₇H₆Cl₂O and a molecular weight of 177.04 g/mol. Spectroscopic studies using ¹H NMR have revealed key structural information about DCA. Research indicates that the minimum energy conformation of DCA involves the chlorine atoms and the methoxy group (–OCH₃) preferentially occupying positions on the plane of the aromatic ring, while the COC and phenyl planes remain orthogonal [, ]. Additionally, the dihedral angle (HCH) within the methyl group of DCA has been determined to be 110.0°, with a C–H bond length (rCH) of 1.14 ± 0.04 Å []. Further analysis using ²H NMR on deuterated DCA (OCD₃) determined the quadrupole coupling constant of the C–D bond to be 144 ± 24 kHz [].
Q2: How stable is this compound under different conditions?
A2: While the provided research does not offer specific data on DCA's stability under various conditions, one study used electron beam irradiation to potentially degrade 2,4,6-trichloroanisole, a related compound often found alongside DCA []. This suggests that DCA, similar to other haloanisoles, might be susceptible to degradation by certain treatments, but further research is needed to confirm this.
Q3: What are the common analytical methods used to detect and quantify this compound?
A3: Gas chromatography coupled with mass spectrometry (GC/MS) is a widely used technique for analyzing this compound, particularly in the context of wine contamination []. This method offers high sensitivity and selectivity for detecting and quantifying DCA, even at trace levels [, ]. Researchers often employ a polydeuterated form of TCA as an internal standard for accurate quantification []. Additionally, headspace solid-phase microextraction (HS-SPME) coupled with GC and a two-dimensional detection system using both an electron capture detector (ECD) and inductively coupled plasma mass spectrometer (ICP-MS) has proven effective in analyzing DCA in complex matrices like wine []. This method combines the halogen sensitivity of ECD with the selectivity of ICP-MS for reliable identification and quantification [].
Q4: How is this compound typically synthesized?
A4: One reported method for synthesizing this compound involves using dimethyl carbonate (DMC) as a methylating agent []. This method utilizes 2,6-dichlorophenol as the starting material and employs potassium carbonate (K₂CO₃) and polyethylene glycol 400 (PEG-400) as a phase-transfer catalyst []. This specific synthesis route offers an alternative to traditional methods that rely on more toxic methylating agents.
Avertissement et informations sur les produits de recherche in vitro
Veuillez noter que tous les articles et informations sur les produits présentés sur BenchChem sont destinés uniquement à des fins informatives. Les produits disponibles à l'achat sur BenchChem sont spécifiquement conçus pour des études in vitro, qui sont réalisées en dehors des organismes vivants. Les études in vitro, dérivées du terme latin "in verre", impliquent des expériences réalisées dans des environnements de laboratoire contrôlés à l'aide de cellules ou de tissus. Il est important de noter que ces produits ne sont pas classés comme médicaments et n'ont pas reçu l'approbation de la FDA pour la prévention, le traitement ou la guérison de toute condition médicale, affection ou maladie. Nous devons souligner que toute forme d'introduction corporelle de ces produits chez les humains ou les animaux est strictement interdite par la loi. Il est essentiel de respecter ces directives pour assurer la conformité aux normes légales et éthiques en matière de recherche et d'expérimentation.