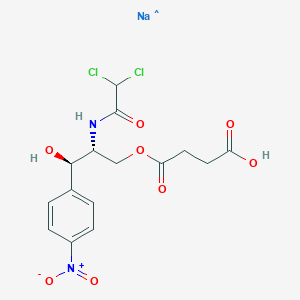
Chloramphénicol sodique succinate
Vue d'ensemble
Description
Succinate de chloramphénicol sodique: est un antibiotique à large spectre utilisé pour traiter les infections bactériennes graves. C'est un promédicament ester de la chloramphénicol, ce qui signifie qu'il est converti en sa forme active, la chloramphénicol, dans l'organisme. Le succinate de chloramphénicol sodique est particulièrement utile pour l'administration intraveineuse en raison de sa solubilité accrue par rapport à la chloramphénicol .
Mécanisme D'action
Target of Action
Chloramphenicol sodium succinate is a prodrug of chloramphenicol . The primary target of chloramphenicol is the bacterial ribosome . Specifically, it binds to the residues A2451 and A2452 in the 23S rRNA of the 50S ribosomal subunit of E. coli . This interaction inhibits bacterial protein synthesis, making chloramphenicol a bacteriostatic antibiotic .
Mode of Action
Chloramphenicol sodium succinate is hydrolyzed into the active chloramphenicol . Chloramphenicol resembles uridine-5’-phosphate . It binds to the bacterial ribosome, blocking the peptidyl transferase step, and preventing the attachment of aminoacyl tRNA to the ribosome . This interaction inhibits bacterial protein synthesis .
Biochemical Pathways
The propane-diol moiety of chloramphenicol can be metabolized through a number of pathways including glucuronidation, sulfate conjugation, phosphorylation, acetylation, and oxidation . The dichloroacetate moiety can be metabolized through hydrolysis of the amide group and dechlorination . The nitro functional group can also be metabolized to an aryl amine and aryl amide metabolite .
Pharmacokinetics
Chloramphenicol succinate acts as a prodrug, being converted to active chloramphenicol while it is circulating in the body . The bioavailability of chloramphenicol after intravenous administration of the succinate ester averages approximately 70%, but the range is quite variable . Incomplete bioavailability is the result of renal excretion of unchanged chloramphenicol succinate prior to it being hydrolyzed to active chloramphenicol . Plasma protein binding of chloramphenicol is approximately 60% in healthy adults . The drug is extensively distributed to many tissues and body fluids, including cerebrospinal fluid and breast milk, and it crosses the placenta . Most of a chloramphenicol dose is metabolized by the liver to inactive products, the chief metabolite being a glucuronide conjugate; only 5 to 15% of chloramphenicol is excreted unchanged in the urine . The elimination half-life is approximately 4 hours .
Result of Action
The result of chloramphenicol’s action is the inhibition of bacterial growth . By binding to the bacterial ribosome and inhibiting protein synthesis, chloramphenicol prevents the bacteria from producing essential proteins, thereby stopping their growth .
Action Environment
The action of chloramphenicol sodium succinate can be influenced by environmental factors. For example, the penetration of chloramphenicol in HeLa 3D tumor sphere model was enhanced, which was related to deeply penetrated microemulsion of small size mediated at the tumor site
Applications De Recherche Scientifique
Chemistry: Chloramphenicol sodium succinate is used in molecular biology for bacterial selection in genetic experiments. It is also employed in the synthesis of various derivatives for research purposes .
Biology: In biological research, chloramphenicol sodium succinate is used to study bacterial protein synthesis and the mechanisms of antibiotic resistance .
Medicine: Clinically, chloramphenicol sodium succinate is used to treat severe infections caused by susceptible bacteria, including meningitis, typhoid fever, and rickettsial infections .
Industry: In the pharmaceutical industry, chloramphenicol sodium succinate is used in the formulation of injectable antibiotics due to its solubility and stability .
Analyse Biochimique
Biochemical Properties
Chloramphenicol sodium succinate is hydrolyzed into the active form, chloramphenicol, in the body . Chloramphenicol binds to bacterial ribosomes and prevents translation . It resembles uridine-5’-phosphate and binds to the residues A2451 and A2452 in the 23S rRNA of the 50S ribosomal subunit of E. coli, which prevents translation .
Cellular Effects
Chloramphenicol sodium succinate, once converted to chloramphenicol, has a broad-spectrum antibiotic effect. It inhibits bacterial protein synthesis by binding to the bacterial ribosome and preventing translation . This action can affect various types of cells and cellular processes, including cell signaling pathways, gene expression, and cellular metabolism.
Molecular Mechanism
The molecular mechanism of action of Chloramphenicol sodium succinate involves its conversion to Chloramphenicol, which then binds to bacterial ribosomes and inhibits protein synthesis . Chloramphenicol binds to the residues A2451 and A2452 in the 23S rRNA of the 50S ribosomal subunit of E. coli, preventing translation .
Temporal Effects in Laboratory Settings
The effects of Chloramphenicol sodium succinate change over time in laboratory settings. It is hydrolyzed into the active form, Chloramphenicol, in the body . The bioavailability of Chloramphenicol after intravenous administration of the succinate ester averages approximately 70%, but the range is quite variable .
Dosage Effects in Animal Models
The effects of Chloramphenicol sodium succinate vary with different dosages in animal models .
Metabolic Pathways
Chloramphenicol sodium succinate is metabolized (hydrolyzed) by esterases in the body to active Chloramphenicol . Most of a Chloramphenicol dose is metabolized by the liver to inactive products, the chief metabolite being a glucuronide conjugate .
Transport and Distribution
Chloramphenicol sodium succinate, once converted to Chloramphenicol, is extensively distributed to many tissues and body fluids, including cerebrospinal fluid and breast milk, and it crosses the placenta .
Subcellular Localization
Chloramphenicol sodium succinate, once converted to Chloramphenicol, is a small molecule that diffuses well into many body tissues including cerebral spinal fluid (CSF) - even in the absence of inflamed meninges, eye, pleural fluid, synovial fluid, ascitic fluid, liver, and kidneys .
Méthodes De Préparation
Voies de synthèse et conditions de réaction: Le succinate de chloramphénicol sodique est synthétisé par estérification de la chloramphénicol avec l'anhydride succinique en présence d'un catalyseur d'amine organique. La réaction implique la formation d'une liaison ester entre le groupe hydroxyle de la chloramphénicol et le groupe carboxyle de l'anhydride succinique .
Méthodes de production industrielle: Dans les milieux industriels, le succinate de chloramphénicol sodique est produit en dissolvant la chloramphénicol dans un solvant approprié et en la faisant réagir avec l'anhydride succinique dans des conditions contrôlées. Le produit est ensuite purifié et converti en sa forme de sel de sodium pour un usage pharmaceutique .
Analyse Des Réactions Chimiques
Types de réactions: Le succinate de chloramphénicol sodique subit une hydrolyse pour libérer la chloramphénicol active. Cette hydrolyse est catalysée par les estérases dans l'organisme .
Réactifs et conditions courants:
Hydrolyse: L'eau et les estérases sont les principaux réactifs impliqués dans l'hydrolyse du succinate de chloramphénicol sodique.
Oxydation et réduction: Le succinate de chloramphénicol sodique ne subit généralement pas de réactions d'oxydation ou de réduction dans des conditions physiologiques.
Principaux produits: Le principal produit de l'hydrolyse est la chloramphénicol, qui exerce l'effet antibiotique en inhibant la synthèse des protéines bactériennes .
Applications de la recherche scientifique
Chimie: Le succinate de chloramphénicol sodique est utilisé en biologie moléculaire pour la sélection bactérienne dans les expériences génétiques. Il est également utilisé dans la synthèse de divers dérivés à des fins de recherche .
Biologie: En recherche biologique, le succinate de chloramphénicol sodique est utilisé pour étudier la synthèse des protéines bactériennes et les mécanismes de résistance aux antibiotiques .
Médecine: Cliniquement, le succinate de chloramphénicol sodique est utilisé pour traiter les infections graves causées par des bactéries sensibles, notamment la méningite, la fièvre typhoïde et les infections rickettsiennes .
Industrie: Dans l'industrie pharmaceutique, le succinate de chloramphénicol sodique est utilisé dans la formulation d'antibiotiques injectables en raison de sa solubilité et de sa stabilité .
Mécanisme d'action
Le succinate de chloramphénicol sodique est hydrolysé en chloramphénicol, qui se lie à la sous-unité ribosomale 50S des ribosomes bactériens. Cette liaison inhibe l'activité de la peptidyl transférase, empêchant la formation de liaisons peptidiques pendant la synthèse des protéines. En conséquence, la croissance bactérienne est stoppée .
Comparaison Avec Des Composés Similaires
Composés similaires:
Chloramphénicol: La forme active du succinate de chloramphénicol sodique, utilisée pour l'administration orale et topique.
Palmitate de chloramphénicol: Un promédicament ester de la chloramphénicol utilisé pour l'administration orale.
Unicité: Le succinate de chloramphénicol sodique est unique en raison de sa solubilité et de sa convenance pour l'administration intraveineuse. Contrairement au palmitate de chloramphénicol, qui est utilisé par voie orale, le succinate de chloramphénicol sodique est préféré pour les infections graves nécessitant une administration rapide et contrôlée de l'antibiotique .
Propriétés
Numéro CAS |
982-57-0 |
---|---|
Formule moléculaire |
C15H16Cl2N2O8.Na C15H16Cl2N2NaO8 |
Poids moléculaire |
446.2 g/mol |
Nom IUPAC |
sodium;4-[(2R,3R)-2-[(2,2-dichloroacetyl)amino]-3-hydroxy-3-(4-nitrophenyl)propoxy]-4-oxobutanoate |
InChI |
InChI=1S/C15H16Cl2N2O8.Na/c16-14(17)15(24)18-10(7-27-12(22)6-5-11(20)21)13(23)8-1-3-9(4-2-8)19(25)26;/h1-4,10,13-14,23H,5-7H2,(H,18,24)(H,20,21);/t10-,13-;/m1./s1 |
Clé InChI |
OQAWGHRXAVKAIG-HTMVYDOJSA-N |
SMILES |
C1=CC(=CC=C1C(C(COC(=O)CCC(=O)[O-])NC(=O)C(Cl)Cl)O)[N+](=O)[O-].[Na+] |
SMILES isomérique |
C1=CC(=CC=C1[C@H]([C@@H](COC(=O)CCC(=O)O)NC(=O)C(Cl)Cl)O)[N+](=O)[O-].[Na] |
SMILES canonique |
C1=CC(=CC=C1C(C(COC(=O)CCC(=O)O)NC(=O)C(Cl)Cl)O)[N+](=O)[O-].[Na] |
Apparence |
White solid |
Key on ui other cas no. |
982-57-0 |
Description physique |
Chloramphenicol sodium succinate is a white powder. (NTP, 1992) |
Pictogrammes |
Irritant; Health Hazard |
Numéros CAS associés |
3544-94-3 (Parent) |
Solubilité |
greater than or equal to 100 mg/mL at 68.9 °F (NTP, 1992) |
Synonymes |
1-[(2R,3R)-2-[(2,2-Dichloroacetyl)amino]-3-hydroxy-3-(4-nitrophenyl)propyl] Ester Butanedioic Acid Sodium Salt; Mono[(2R,3R)-2-[(dichloroacetyl)amino]-3-hydroxy-3-(4-nitrophenyl)propyl] Ester Butanedioic Acid Monosodium Salt; Betamicetin; Chloramphen |
Origine du produit |
United States |
Retrosynthesis Analysis
AI-Powered Synthesis Planning: Our tool employs the Template_relevance Pistachio, Template_relevance Bkms_metabolic, Template_relevance Pistachio_ringbreaker, Template_relevance Reaxys, Template_relevance Reaxys_biocatalysis model, leveraging a vast database of chemical reactions to predict feasible synthetic routes.
One-Step Synthesis Focus: Specifically designed for one-step synthesis, it provides concise and direct routes for your target compounds, streamlining the synthesis process.
Accurate Predictions: Utilizing the extensive PISTACHIO, BKMS_METABOLIC, PISTACHIO_RINGBREAKER, REAXYS, REAXYS_BIOCATALYSIS database, our tool offers high-accuracy predictions, reflecting the latest in chemical research and data.
Strategy Settings
Precursor scoring | Relevance Heuristic |
---|---|
Min. plausibility | 0.01 |
Model | Template_relevance |
Template Set | Pistachio/Bkms_metabolic/Pistachio_ringbreaker/Reaxys/Reaxys_biocatalysis |
Top-N result to add to graph | 6 |
Feasible Synthetic Routes
A: Chloramphenicol sodium succinate is a prodrug that is hydrolyzed in vivo to Chloramphenicol. [] Chloramphenicol acts by reversibly binding to the 50S subunit of bacterial ribosomes, specifically targeting the peptidyl transferase activity. [] This binding interferes with the elongation process of protein synthesis, effectively preventing bacterial cell growth. []
ANone: While a detailed spectroscopic analysis is not provided in these abstracts, Chloramphenicol sodium succinate is the sodium succinate ester of Chloramphenicol. This means a succinic acid moiety is attached to the Chloramphenicol molecule, and the carboxyl group of succinic acid is present in its sodium salt form.
A: Research suggests Chloramphenicol sodium succinate can be loaded onto silicone hydrogel contact lenses, demonstrating potential for sustained release in treating ocular bacterial infections. [] The drug release was found to be directly proportional to the loading concentration. []
ANone: Chloramphenicol sodium succinate is not known to possess catalytic properties. Its primary application stems from the antibacterial activity of its active metabolite, Chloramphenicol.
ANone: The provided abstracts do not delve into computational chemistry studies or QSAR models for Chloramphenicol sodium succinate.
A: Information regarding the stability of different Chloramphenicol sodium succinate formulations is limited in the abstracts. One study mentions that Chloramphenicol sodium succinate can be hydrolyzed to active Chloramphenicol in ventricular fluid. []
ANone: The provided research abstracts do not specifically address SHE regulations concerning Chloramphenicol sodium succinate.
A: Chloramphenicol sodium succinate, when administered intramuscularly, is well absorbed and achieves therapeutic levels in the blood. [] Studies in children showed peak blood levels comparable to those achieved with intravenous administration, suggesting efficient absorption from muscle tissue. []
A: In a study on adult horses, the mean bioavailability of Chloramphenicol base after oral administration was found to be 28%, significantly lower than the bioavailability achieved with intravenous Chloramphenicol sodium succinate. []
A: Age significantly impacts the pharmacokinetics of Chloramphenicol in foals. Studies show that elimination half-life (t1/2 beta) decreases significantly from 5.29 hours in one-day-old foals to 0.34 hours in 42-day-old foals, indicating faster clearance with increasing age. [] This highlights the importance of age-specific dosing regimens for this drug in horses.
A: Hemodialysis can significantly increase the total body clearance (ClTB) of Chloramphenicol, especially in patients with compromised liver function. [] This increase in clearance during dialysis necessitates careful monitoring of drug levels and potential dose adjustments. []
A: Studies suggest that treatment of bacterial meningitis in children with intramuscular Chloramphenicol sodium succinate, combined with oral probenecid and chloramphenicol palmitate, can be as effective as intravenous administration of Chloramphenicol. [] This suggests that alternative routes of administration can be considered for this condition.
A: While the provided abstracts do not extensively cover resistance mechanisms, one study investigating Haemophilus species bacteremia in adults highlights a concerning trend of increasing resistance to Chloramphenicol. [] This underscores the importance of monitoring resistance patterns and adapting treatment strategies accordingly.
A: One study explored the possibility of using silicone hydrogel contact lenses as a sustained-release platform for Chloramphenicol sodium succinate in treating ocular infections. [] This approach holds promise for improving patient compliance and achieving targeted drug delivery to the eye.
ANone: The provided abstracts do not discuss specific biomarkers for predicting efficacy, monitoring treatment response, or identifying adverse effects associated with Chloramphenicol sodium succinate.
ANone: Several analytical methods are mentioned in the research for measuring Chloramphenicol and its metabolites in various biological matrices:
- High-Performance Liquid Chromatography (HPLC): Utilized for determining Chloramphenicol concentrations in plasma and milk. [, , , ]
- Microbiological Assay: Used to determine plasma chloramphenicol concentrations. []
- Chloramphenicol-ELISA: Employed for monitoring serum chloramphenicol concentrations. []
- Chemical Colorimetric Method: Used to quantify plasma chloramphenicol levels. []
A: Yes, radial-compression liquid chromatography allows for the direct and simultaneous measurement of Chloramphenicol and its monosuccinate ester in minute plasma samples (as small as 10 μL). [] This method offers a rapid and efficient way to analyze both compounds in clinical settings.
ANone: The environmental impact and degradation of Chloramphenicol sodium succinate are not discussed in the provided abstracts.
ANone: The provided abstracts do not discuss studies specifically addressing the dissolution rate or solubility of Chloramphenicol sodium succinate in various media.
A: While not extensively detailed, some abstracts mention validation parameters like accuracy, precision, and specificity for the employed analytical methods, emphasizing the importance of reliable and robust analytical techniques. [, ]
ANone: The research papers provided do not delve into the specifics of quality control and assurance measures during the development, manufacturing, or distribution of Chloramphenicol sodium succinate.
ANone: The abstracts do not discuss the potential of Chloramphenicol sodium succinate to induce an immune response or any strategies to address immunogenicity concerns.
ANone: The research provided does not provide information on interactions between Chloramphenicol sodium succinate and drug transporters.
A: Contrary to its effects observed in other species, Chloramphenicol, even at doses causing a significant decrease in the activity of hepatic biotransformation enzymes (aniline hydroxylase and ethylmorphine N-demethylase), did not prolong the duration of pentobarbital or ketamine/xylazine anesthesia in guinea pigs. [] This suggests species-specific differences in drug interactions and highlights the importance of considering species-specific metabolism when extrapolating data.
Avertissement et informations sur les produits de recherche in vitro
Veuillez noter que tous les articles et informations sur les produits présentés sur BenchChem sont destinés uniquement à des fins informatives. Les produits disponibles à l'achat sur BenchChem sont spécifiquement conçus pour des études in vitro, qui sont réalisées en dehors des organismes vivants. Les études in vitro, dérivées du terme latin "in verre", impliquent des expériences réalisées dans des environnements de laboratoire contrôlés à l'aide de cellules ou de tissus. Il est important de noter que ces produits ne sont pas classés comme médicaments et n'ont pas reçu l'approbation de la FDA pour la prévention, le traitement ou la guérison de toute condition médicale, affection ou maladie. Nous devons souligner que toute forme d'introduction corporelle de ces produits chez les humains ou les animaux est strictement interdite par la loi. Il est essentiel de respecter ces directives pour assurer la conformité aux normes légales et éthiques en matière de recherche et d'expérimentation.