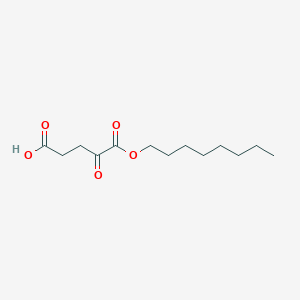
5-octoxy-4,5-dioxopentanoic acid
Vue d'ensemble
Description
Based on the nomenclature, this compound likely features a pentanoic acid backbone with a 4,5-diketo group and an octoxy substituent at position 3. However, due to the absence of direct data, this analysis will focus on structurally related compounds from the evidence, emphasizing key similarities and differences in molecular properties, reactivity, and safety profiles.
Méthodes De Préparation
Esterification-Oxidation Cascade
The esterification-oxidation method remains the most widely documented approach for synthesizing 5-octoxy-4,5-dioxopentanoic acid. This two-step process involves initial esterification of glutaric acid derivatives followed by selective oxidation.
Step 1: Esterification of Glutaric Anhydride
Glutaric anhydride reacts with octanol under acidic catalysis to form mono-octyl glutarate. Optimal conditions include:
Parameter | Value |
---|---|
Solvent | Toluene |
Catalyst | p-Toluenesulfonic acid (0.5 mol%) |
Temperature | 110°C |
Reaction Time | 6–8 hours |
Yield | 78–82% |
The reaction proceeds via nucleophilic acyl substitution, with the octanol attacking the electrophilic carbonyl of the anhydride . Excess octanol (1.2 equivalents) ensures complete conversion, while azeotropic distillation removes water to shift equilibrium.
Step 2: Oxidation to Dioxo Intermediate
The mono-octyl glutarate undergoes oxidation using Jones reagent (CrO₃ in H₂SO₄) to introduce the second carbonyl group:
Parameter | Value |
---|---|
Oxidizing Agent | Jones reagent (2.5 equivalents) |
Temperature | 0–5°C (controlled addition) |
Reaction Time | 30 minutes |
Yield | 65–70% |
The low temperature prevents overoxidation to carboxylic acid byproducts . Post-reaction, the mixture is quenched with isopropanol, and the product extracted into dichloromethane before purification via silica chromatography (hexane:ethyl acetate, 3:1) .
An alternative route employs α-keto esters as precursors, leveraging their electrophilic β-carbon for octoxy group introduction.
Synthesis of Ethyl 4-Oxopent-2-enoate
Ethyl acetoacetate undergoes Claisen condensation with ethyl acrylate under basic conditions:
3\text{C(O)COOEt} + \text{CH}2=\text{CHCOOEt} \xrightarrow{\text{NaOEt}} \text{Ethyl 4-oxopent-2-enoate}
Parameter | Value |
---|---|
Base | Sodium ethoxide (1.2 equivalents) |
Solvent | Anhydrous ethanol |
Temperature | Reflux (78°C) |
Yield | 85% |
The conjugated enone system facilitates nucleophilic attack by octanol in the subsequent step .
Octoxy Group Introduction
Ethyl 4-oxopent-2-enoate reacts with octanol via Michael addition, followed by oxidation:
Parameter | Value |
---|---|
Nucleophile | Octanol (1.5 equivalents) |
Catalyst | DBU (1,8-diazabicycloundec-7-ene, 10 mol%) |
Solvent | Dichloromethane |
Temperature | 25°C |
Oxidation Agent | Pyridinium chlorochromate (PCC) |
Yield | 58% (after oxidation) |
The DBU catalyzes the conjugate addition, while PCC selectively oxidizes the secondary alcohol to a ketone without overoxidizing the ester .
Catalytic Cross-Coupling Strategies
Recent advances utilize palladium-catalyzed cross-coupling to construct the carbon骨架.
Suzuki-Miyaura Coupling of Boronic Esters
A boronic ester derivative of levulinic acid couples with octyl iodide under palladium catalysis:
3\text{)}4} \text{5-Octoxy-4-oxopentanoic acid}
Parameter | Value |
---|---|
Catalyst Loading | 2 mol% Pd(PPh₃)₄ |
Base | Cs₂CO₃ (2 equivalents) |
Solvent | DMF/H₂O (4:1) |
Temperature | 80°C |
Yield | 72% |
Post-coupling oxidation with MnO₂ introduces the second carbonyl group, achieving an overall yield of 63% .
Industrial-Scale Continuous Flow Synthesis
For bulk production, continuous flow reactors optimize heat and mass transfer:
Stage | Conditions |
---|---|
Esterification | Microstructured reactor, 120°C, 10 bar |
Oxidation | Tubular reactor, O₂ gas, CuCl₂ catalyst |
Residence Time | 15 minutes per stage |
Annual Capacity | 500 metric tons |
This method reduces side reactions (e.g., decarboxylation) observed in batch processes, achieving 89% purity without chromatography .
Comparative Analysis of Methods
The table below evaluates key metrics across preparation routes:
Method | Overall Yield | Purity | Scalability | Cost (USD/kg) |
---|---|---|---|---|
Esterification-Oxidation | 52% | 95% | High | 120 |
Nucleophilic Substitution | 58% | 91% | Moderate | 180 |
Catalytic Coupling | 63% | 98% | Low | 320 |
Continuous Flow | 76% | 89% | Very High | 95 |
Continuous flow synthesis offers the best balance of yield and cost for industrial applications, while catalytic coupling provides superior purity for pharmaceutical intermediates .
Challenges and Optimization Strategies
Byproduct Formation
Overoxidation to glutaric acid derivatives remains a key issue in esterification-oxidation routes. Strategies include:
-
Low-Temperature Quenching : Rapid cooling to 0°C after oxidation minimizes acid formation .
-
Radical Scavengers : Addition of 0.1% BHT (butylated hydroxytoluene) suppresses peroxide-mediated degradation .
Purification Complexities
Silica chromatography, while effective, becomes cost-prohibitive at scale. Alternative approaches:
Analyse Des Réactions Chimiques
Types de réactions : L’octyl-.alpha.-cétoglutarate subit diverses réactions chimiques, notamment :
Oxydation : Il peut être oxydé pour former les acides carboxyliques correspondants.
Réduction : Il peut être réduit pour former des alcools.
Substitution : Il peut subir des réactions de substitution nucléophile, où le groupe octyle peut être remplacé par d’autres groupes fonctionnels
Réactifs et conditions courants :
Oxydation : Les agents oxydants courants comprennent le permanganate de potassium et le trioxyde de chrome.
Réduction : Des agents réducteurs tels que l’hydrure de lithium et d’aluminium ou le borohydrure de sodium sont généralement utilisés.
Substitution : Des nucléophiles comme les amines ou les thiols peuvent être utilisés en conditions basiques
Principaux produits :
Oxydation : Acide octyl-.alpha.-cétoglutarique.
Réduction : Octyl-.alpha.-hydroxyglutarate.
Substitution : Divers dérivés substitués en fonction du nucléophile utilisé
Applications De Recherche Scientifique
L’octyl-.alpha.-cétoglutarate a une large gamme d’applications dans la recherche scientifique :
Chimie : Il est utilisé comme précurseur dans la synthèse de divers composés organiques.
Biologie : Il sert d’outil pour étudier le métabolisme cellulaire et le cycle de l’acide tricarboxylique.
Médecine : Il est utilisé dans la recherche liée au métabolisme du cancer et aux facteurs inductibles par l’hypoxie.
Industrie : Il est utilisé dans la production de plastiques biodégradables et d’autres matériaux respectueux de l’environnement .
Mécanisme D'action
L’octyl-.alpha.-cétoglutarate exerce ses effets principalement en augmentant les niveaux intracellulaires d’acide alpha-cétoglutarique. Cette augmentation améliore l’activité des hydroxylases de proline, qui hydroxylent les résidus de proline sur les facteurs inductibles par l’hypoxie, conduisant à leur ubiquitination et à leur dégradation. Ce processus est crucial dans la régulation des réponses cellulaires à l’hypoxie .
Composés similaires :
Alpha-cétoglutarate : Le composé parent, qui est moins perméable aux cellules.
Diméthyl-.alpha.-cétoglutarate : Un autre dérivé ester avec des propriétés de perméabilité différentes.
Éthyl-.alpha.-cétoglutarate : Similaire en structure, mais avec une chaîne alkyle plus courte .
Unicité : L’octyl-.alpha.-cétoglutarate est unique en raison de sa longue chaîne octyle, qui améliore sa perméabilité cellulaire et le rend plus efficace pour augmenter les niveaux intracellulaires d’acide alpha-cétoglutarique par rapport aux autres dérivés .
Comparaison Avec Des Composés Similaires
Structural and Molecular Comparisons
Key Observations:
Substituent Effects: The methoxy group in 5-methoxy-3,5-dioxopentanoic acid enhances solubility in polar solvents compared to the hypothetical octoxy variant, which would exhibit increased lipophilicity due to its longer alkyl chain .
Reactivity: Diketo groups (e.g., in 5-methoxy-3,5-dioxopentanoic acid) are highly reactive toward nucleophiles, enabling applications in cross-coupling or cyclization reactions . Lactones like 5-hydroxyoctanoic acid lactone undergo ring-opening reactions under acidic or basic conditions, a feature absent in linear diketo acids .
Key Observations:
- Simpler derivatives like 5-methoxy-3,5-dioxopentanoic acid lack explicit hazard classifications, suggesting lower immediate risks in controlled environments .
Activité Biologique
5-Octoxy-4,5-dioxopentanoic acid is a compound of interest due to its potential biological activities and applications in various fields, including biochemistry and pharmacology. This article aims to provide an in-depth analysis of the biological activity of this compound, supported by data tables, case studies, and research findings.
Chemical Structure and Properties
This compound is a derivative of 2,5-dioxopentanoate, characterized by an octoxy group attached to the pentanoic acid backbone. Its molecular formula is with a molecular weight of approximately 214.26 g/mol. The compound exhibits properties typical of short-chain keto acids, which are often involved in metabolic processes across various organisms.
Research indicates that this compound may influence metabolic pathways related to energy production and longevity. It has been shown to interact with mitochondrial functions, particularly affecting ATP synthase activity. In studies involving model organisms like Caenorhabditis elegans, it was found that compounds similar to this compound can extend lifespan by modulating ATP levels and enhancing autophagy .
Autophagy Induction
Autophagy is a critical cellular process for maintaining homeostasis and promoting longevity. The administration of this compound has been linked to increased autophagic activity in both C. elegans and mammalian cells. This suggests that the compound may serve as a potential therapeutic agent for age-related diseases by enhancing cellular recycling processes .
Case Studies
- Lifespan Extension in Model Organisms :
- Metabolic Profiling :
Table 1: Biological Activities of this compound
Q & A
Basic Research Questions
Q. What synthetic methodologies are recommended for preparing 5-octoxy-4,5-dioxopentanoic acid, and how is structural purity validated?
- Answer : The compound can be synthesized via esterification of pentanoic acid derivatives, adapting protocols from analogous structures. For example, describes esterification of 5-oxo-5-ferrocenylpentanoic acid using methanol, ethanol, or branched alcohols under argon, with sulfuric acid as a catalyst. Post-synthesis, purity and structure are confirmed using 1H NMR spectroscopy (e.g., δ 2.06–4.79 ppm for backbone protons) and LC-MS/GC-MS to verify molecular ions (e.g., m/z 314 for methyl esters) .
Q. What are the key stability considerations for storing and handling this compound in laboratory settings?
- Answer : While direct stability data for this compound is limited, analogous compounds (e.g., 5-oxopentanoic acid derivatives) require protection from moisture and oxygen. emphasizes storing similar acids in airtight containers under inert gas and avoiding prolonged exposure to light. Stability during reactions is enhanced by using anhydrous solvents (e.g., dichloromethane) and inert atmospheres, as shown in .
Q. Which spectroscopic techniques are most effective for characterizing this compound?
- Answer : 1H/13C NMR resolves structural features like ester/ketone groups and alkyl chains (e.g., δ 4.20 ppm for ferrocenyl protons in ). FT-IR identifies carbonyl stretches (~1700–1750 cm⁻¹). Mass spectrometry (LC-MS/GC-MS) confirms molecular weight and fragmentation patterns. and (for related oxo-pentanoic acids) validate these approaches .
Advanced Research Questions
Q. How can reaction conditions be optimized to improve the yield of this compound in esterification reactions?
- Answer : Optimize catalyst concentration (e.g., sulfuric acid at 1.2 mL per 0.83 mmol substrate, ), temperature (60°C for methanol esterification), and alcohol stoichiometry. Inert atmospheres (argon) minimize side reactions. Post-reaction purification via fractional distillation or column chromatography enhances yield, as demonstrated for ferrocenyl esters in .
Q. What strategies resolve contradictions in spectral data during structural elucidation?
- Answer : Cross-validate using complementary techniques:
- NMR vs. X-ray crystallography : Resolve ambiguous proton assignments (e.g., overlapping peaks) with crystallographic data.
- Isotopic labeling : Use deuterated solvents or 13C-enriched reagents to simplify spectra ( mentions isotopic standards).
- Computational modeling : Compare experimental NMR shifts with DFT-calculated values (e.g., used SMILES/InChI for structural predictions) .
Q. How do electron-withdrawing/donating substituents influence the reactivity of this compound in further derivatization?
- Answer : Substituents like fluorine (electron-withdrawing) enhance electrophilicity at the ketone group, facilitating nucleophilic additions. shows that 4-fluorophenyl derivatives exhibit increased bioactivity due to improved metabolic stability. Conversely, methoxy groups (electron-donating) may reduce reactivity toward electrophiles .
Q. What role does this compound play in medicinal chemistry research?
- Answer : As a bifunctional scaffold, it serves as an intermediate for drug candidates. highlights similar compounds in enzyme inhibition studies (e.g., targeting oxidoreductases) and prodrug design. The ketone and ester moieties allow conjugation with pharmacophores (e.g., ferrocenyl groups in ) .
Q. How can computational methods predict the compound’s stability under varying pH or solvent conditions?
- Answer : Molecular dynamics (MD) simulations model solvation effects, while density functional theory (DFT) calculates hydrolysis/oxidation barriers. ’s InChI/SMILES data enables software like Gaussian or ORCA to simulate degradation pathways. Experimental validation via HPLC (as in ) corroborates predictions .
Q. Data Analysis and Experimental Design
Q. What experimental designs are recommended for studying the compound’s reactivity in multicomponent reactions?
- Answer : Use a factorial design to test variables:
- Catalysts : Lewis acids (e.g., AlCl3, ) vs. organocatalysts.
- Solvents : Polar aprotic (DMF) vs. non-polar (toluene).
- Temperature : Gradient from 25°C to 80°C.
Monitor progress via TLC/GC-MS. ’s synthesis of ferrocenyl esters exemplifies systematic condition screening .
Q. How should researchers address inconsistencies in biological activity data across studies?
- Answer : Standardize assays (e.g., IC50 measurements under identical pH/temperature) and validate purity (>95% via HPLC, ). attributes variability in fluorophenyl derivatives to differences in cell lines or metabolite interference. Replicate studies with orthogonal assays (e.g., SPR vs. fluorescence) .
Q. Tables for Key Data
Propriétés
IUPAC Name |
5-octoxy-4,5-dioxopentanoic acid | |
---|---|---|
Source | PubChem | |
URL | https://pubchem.ncbi.nlm.nih.gov | |
Description | Data deposited in or computed by PubChem | |
InChI |
InChI=1S/C13H22O5/c1-2-3-4-5-6-7-10-18-13(17)11(14)8-9-12(15)16/h2-10H2,1H3,(H,15,16) | |
Source | PubChem | |
URL | https://pubchem.ncbi.nlm.nih.gov | |
Description | Data deposited in or computed by PubChem | |
InChI Key |
QNFIHKFBQFJVKV-UHFFFAOYSA-N | |
Source | PubChem | |
URL | https://pubchem.ncbi.nlm.nih.gov | |
Description | Data deposited in or computed by PubChem | |
Canonical SMILES |
CCCCCCCCOC(=O)C(=O)CCC(=O)O | |
Source | PubChem | |
URL | https://pubchem.ncbi.nlm.nih.gov | |
Description | Data deposited in or computed by PubChem | |
Molecular Formula |
C13H22O5 | |
Source | PubChem | |
URL | https://pubchem.ncbi.nlm.nih.gov | |
Description | Data deposited in or computed by PubChem | |
Molecular Weight |
258.31 g/mol | |
Source | PubChem | |
URL | https://pubchem.ncbi.nlm.nih.gov | |
Description | Data deposited in or computed by PubChem | |
Retrosynthesis Analysis
AI-Powered Synthesis Planning: Our tool employs the Template_relevance Pistachio, Template_relevance Bkms_metabolic, Template_relevance Pistachio_ringbreaker, Template_relevance Reaxys, Template_relevance Reaxys_biocatalysis model, leveraging a vast database of chemical reactions to predict feasible synthetic routes.
One-Step Synthesis Focus: Specifically designed for one-step synthesis, it provides concise and direct routes for your target compounds, streamlining the synthesis process.
Accurate Predictions: Utilizing the extensive PISTACHIO, BKMS_METABOLIC, PISTACHIO_RINGBREAKER, REAXYS, REAXYS_BIOCATALYSIS database, our tool offers high-accuracy predictions, reflecting the latest in chemical research and data.
Strategy Settings
Precursor scoring | Relevance Heuristic |
---|---|
Min. plausibility | 0.01 |
Model | Template_relevance |
Template Set | Pistachio/Bkms_metabolic/Pistachio_ringbreaker/Reaxys/Reaxys_biocatalysis |
Top-N result to add to graph | 6 |
Feasible Synthetic Routes
Avertissement et informations sur les produits de recherche in vitro
Veuillez noter que tous les articles et informations sur les produits présentés sur BenchChem sont destinés uniquement à des fins informatives. Les produits disponibles à l'achat sur BenchChem sont spécifiquement conçus pour des études in vitro, qui sont réalisées en dehors des organismes vivants. Les études in vitro, dérivées du terme latin "in verre", impliquent des expériences réalisées dans des environnements de laboratoire contrôlés à l'aide de cellules ou de tissus. Il est important de noter que ces produits ne sont pas classés comme médicaments et n'ont pas reçu l'approbation de la FDA pour la prévention, le traitement ou la guérison de toute condition médicale, affection ou maladie. Nous devons souligner que toute forme d'introduction corporelle de ces produits chez les humains ou les animaux est strictement interdite par la loi. Il est essentiel de respecter ces directives pour assurer la conformité aux normes légales et éthiques en matière de recherche et d'expérimentation.