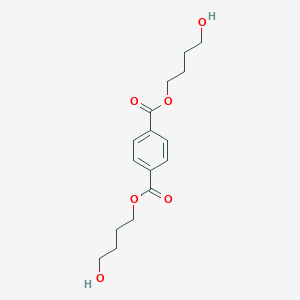
Téréphtalate de bis(4-hydroxybutyle)
Vue d'ensemble
Description
Bis(4-hydroxybutyl) terephthalate is an organic compound with the molecular formula C16H22O6. It is a derivative of terephthalic acid and is characterized by the presence of two 4-hydroxybutyl groups attached to the terephthalate core. This compound is primarily used in the synthesis of polyesters and polyurethanes, contributing to the production of various industrial and commercial materials.
Applications De Recherche Scientifique
Bis(4-hydroxybutyl) terephthalate is utilized in various scientific research domains, including:
Polymer Synthesis: It is a key intermediate in the production of polyesters and polyurethanes, contributing to the development of materials with desirable mechanical properties.
Biomedical Engineering: The compound’s biocompatibility makes it suitable for use in medical devices and implants.
Material Science: Its versatile properties allow for the creation of advanced materials with specific characteristics, such as high tensile strength and thermal stability.
Mécanisme D'action
Target of Action
Bis(4-hydroxybutyl) terephthalate (BHBT) is primarily used in the synthesis of polymers, specifically non-isocyanate thermoplastic polyurethanes (NI-TPUs) . The primary targets of BHBT are the monomers that it interacts with during the polymerization process .
Mode of Action
BHBT is synthesized from the condensation of dimethyl terephthalate with excessive 1,4-butanediol . It then undergoes transurethane polycondensation with 1,6-bis(hydroxyethyloxy carbonyl amino) hexane (BHCH) at different BHCH/BHBT molar ratios . This process is carried out at 170 °C under a reduced pressure of 3 mmHg . The interaction between BHBT and BHCH results in the formation of NI-TPUs .
Biochemical Pathways
The biochemical pathway involved in the action of BHBT is the polymerization process. This process involves the reaction of BHBT with BHCH to form NI-TPUs . The polymerization process is catalyzed by titanium iso-propoxide .
Result of Action
The result of BHBT’s action is the formation of NI-TPUs . These polymers exhibit a number of desirable properties, including a high melting point ™, good tensile strength, and good toughness . The introduction of dibutylene terephthalate units into the polymer structure improves the mechanical properties .
Action Environment
The action of BHBT is influenced by several environmental factors. The temperature and pressure conditions under which the polycondensation reaction occurs are critical for the successful formation of NI-TPUs . Additionally, the presence of a catalyst, such as titanium iso-propoxide, is necessary for the transesterification process .
Méthodes De Préparation
Synthetic Routes and Reaction Conditions: Bis(4-hydroxybutyl) terephthalate is synthesized through the condensation reaction of dimethyl terephthalate with an excess of 1,4-butanediol. The reaction is typically catalyzed by titanium iso-propoxide, which has been found to be more effective than metal acetates . The reaction is carried out at elevated temperatures, around 170°C, under reduced pressure to facilitate the removal of methanol, a by-product of the reaction .
Industrial Production Methods: In industrial settings, the production of bis(4-hydroxybutyl) terephthalate involves the transesterification of bis(2-hydroxyethyl) terephthalate with 1,4-butanediol. Catalysts such as zinc acetate, antimony(III) oxide, tin(II) chloride, and titanium iso-propoxide are used to enhance the reaction efficiency . The reaction conditions, including temperature and catalyst loading, are optimized to achieve high yields and conversion rates.
Analyse Des Réactions Chimiques
Types of Reactions: Bis(4-hydroxybutyl) terephthalate undergoes various chemical reactions, including:
Transesterification: This reaction involves the exchange of ester groups between molecules, often catalyzed by titanium iso-propoxide.
Polycondensation: This reaction leads to the formation of polyesters, such as poly(1,4-butylene terephthalate), through the condensation of bis(4-hydroxybutyl) terephthalate.
Common Reagents and Conditions:
Catalysts: Titanium iso-propoxide, zinc acetate, antimony(III) oxide, tin(II) chloride.
Conditions: Elevated temperatures (170-210°C), reduced pressure, and specific catalyst loadings to optimize reaction efficiency.
Major Products:
Poly(1,4-butylene terephthalate): A thermoplastic polyester with applications in engineering and industrial materials.
Comparaison Avec Des Composés Similaires
Bis(2-hydroxyethyl) terephthalate: Another derivative of terephthalic acid, used in the production of polyesters.
Poly(1,4-butylene terephthalate): A polymer formed from bis(4-hydroxybutyl) terephthalate, known for its engineering applications.
Uniqueness: Bis(4-hydroxybutyl) terephthalate is unique due to its specific structure, which allows for the formation of polyesters with superior mechanical properties. The presence of 4-hydroxybutyl groups enhances the flexibility and toughness of the resulting polymers, making them suitable for a wide range of applications .
Propriétés
IUPAC Name |
bis(4-hydroxybutyl) benzene-1,4-dicarboxylate | |
---|---|---|
Source | PubChem | |
URL | https://pubchem.ncbi.nlm.nih.gov | |
Description | Data deposited in or computed by PubChem | |
InChI |
InChI=1S/C16H22O6/c17-9-1-3-11-21-15(19)13-5-7-14(8-6-13)16(20)22-12-4-2-10-18/h5-8,17-18H,1-4,9-12H2 | |
Source | PubChem | |
URL | https://pubchem.ncbi.nlm.nih.gov | |
Description | Data deposited in or computed by PubChem | |
InChI Key |
MRLFFZIIRRKXBJ-UHFFFAOYSA-N | |
Source | PubChem | |
URL | https://pubchem.ncbi.nlm.nih.gov | |
Description | Data deposited in or computed by PubChem | |
Canonical SMILES |
C1=CC(=CC=C1C(=O)OCCCCO)C(=O)OCCCCO | |
Source | PubChem | |
URL | https://pubchem.ncbi.nlm.nih.gov | |
Description | Data deposited in or computed by PubChem | |
Molecular Formula |
C16H22O6 | |
Source | PubChem | |
URL | https://pubchem.ncbi.nlm.nih.gov | |
Description | Data deposited in or computed by PubChem | |
DSSTOX Substance ID |
DTXSID80451455 | |
Record name | bis(hydroxybutyl) terephthalate | |
Source | EPA DSSTox | |
URL | https://comptox.epa.gov/dashboard/DTXSID80451455 | |
Description | DSSTox provides a high quality public chemistry resource for supporting improved predictive toxicology. | |
Molecular Weight |
310.34 g/mol | |
Source | PubChem | |
URL | https://pubchem.ncbi.nlm.nih.gov | |
Description | Data deposited in or computed by PubChem | |
CAS No. |
23358-95-4 | |
Record name | bis(hydroxybutyl) terephthalate | |
Source | EPA DSSTox | |
URL | https://comptox.epa.gov/dashboard/DTXSID80451455 | |
Description | DSSTox provides a high quality public chemistry resource for supporting improved predictive toxicology. | |
Record name | Bis(4-hydroxybutyl) terephthalate | |
Source | FDA Global Substance Registration System (GSRS) | |
URL | https://gsrs.ncats.nih.gov/ginas/app/beta/substances/LEN57P2BTT | |
Description | The FDA Global Substance Registration System (GSRS) enables the efficient and accurate exchange of information on what substances are in regulated products. Instead of relying on names, which vary across regulatory domains, countries, and regions, the GSRS knowledge base makes it possible for substances to be defined by standardized, scientific descriptions. | |
Explanation | Unless otherwise noted, the contents of the FDA website (www.fda.gov), both text and graphics, are not copyrighted. They are in the public domain and may be republished, reprinted and otherwise used freely by anyone without the need to obtain permission from FDA. Credit to the U.S. Food and Drug Administration as the source is appreciated but not required. | |
Synthesis routes and methods I
Procedure details
Synthesis routes and methods II
Procedure details
Synthesis routes and methods III
Procedure details
Avertissement et informations sur les produits de recherche in vitro
Veuillez noter que tous les articles et informations sur les produits présentés sur BenchChem sont destinés uniquement à des fins informatives. Les produits disponibles à l'achat sur BenchChem sont spécifiquement conçus pour des études in vitro, qui sont réalisées en dehors des organismes vivants. Les études in vitro, dérivées du terme latin "in verre", impliquent des expériences réalisées dans des environnements de laboratoire contrôlés à l'aide de cellules ou de tissus. Il est important de noter que ces produits ne sont pas classés comme médicaments et n'ont pas reçu l'approbation de la FDA pour la prévention, le traitement ou la guérison de toute condition médicale, affection ou maladie. Nous devons souligner que toute forme d'introduction corporelle de ces produits chez les humains ou les animaux est strictement interdite par la loi. Il est essentiel de respecter ces directives pour assurer la conformité aux normes légales et éthiques en matière de recherche et d'expérimentation.