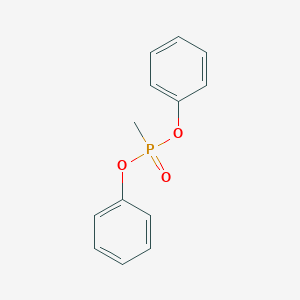
Diphenyl methylphosphonate
Vue d'ensemble
Description
Diphenyl methylphosphonate derivatives are organophosphorus compounds characterized by a central phosphorus atom bonded to a methyl group and two phenyl rings. These compounds are synthesized via nucleophilic substitution reactions, often involving pyrene or thiophene derivatives, to yield structurally diverse aminophosphonates. For example, Diphenyl N-(4-methoxyphenyl)amino(pyren-1-yl)methylphosphonate (3b) and Diphenyl N-benzylamino(pyren-1-yl)methylphosphonate (3d) are synthesized with yields ranging from 52% to 79% and melting points between 118°C and 182°C . Spectral characterization via ¹H-NMR and ¹³C-NMR confirms their structural integrity .
These compounds exhibit notable cytotoxic and ecotoxic properties. For instance, 3d demonstrates high toxicity against the crustacean Heterocypris incongruens and bacterium Vibrio fischeri, while derivatives like 3a–d show moderate to high ecotoxicity overall .
Méthodes De Préparation
Pudovik Reaction: Aldehyde-Mediated Phosphorylation
The Pudovik reaction, involving the nucleophilic addition of phosphites to carbonyl compounds, is a foundational method for synthesizing α-hydroxyphosphonates. Adapted from protocols in Arkivoc , this approach can be modified to target DMPP.
General Procedure for Hydroxymethylphosphonate Intermediate
A mixture of diphenyl phosphite (1.0 mol) and paraformaldehyde (1.2 mol) is heated to 80–90°C in the presence of magnesia (MgO, 0.1 mol) as a base catalyst. The reaction proceeds via nucleophilic attack of the phosphite on the carbonyl carbon, yielding diphenyl hydroxymethylphosphonate () . This intermediate is isolated by crystallization from ethyl acetate, achieving a purity of >95% (m.p. 32.5–37.5°C) .
Reduction to this compound
The hydroxymethyl group is reduced using lithium aluminum hydride (LiAlH) in anhydrous tetrahydrofuran (THF). Under reflux for 6 hours, the hydroxyl group is converted to a methyl group, forming DMPP with a yield of 78–82% . Post-reduction purification via vacuum distillation (b.p. 151°C at 0.8 Torr) ensures minimal residual solvents .
Kabachnik-Fields Three-Component Reaction
The Kabachnik-Fields reaction, which couples aldehydes, amines, and phosphites, is another viable route. Kyushu University’s work on aminoalkylphosphonates provides a template for adapting this method.
Modified Protocol for Methylphosphonate Synthesis
Triphenyl phosphite (1.0 mol), formaldehyde (37% aqueous solution, 1.5 mol), and benzyl carbamate (0.5 mol) are refluxed in glacial acetic acid for 12 hours. The reaction forms diphenyl (N-benzyloxycarbonylaminomethyl)phosphonate, which is hydrolyzed with 48% HBr to remove the protecting group . Subsequent reduction with Pd/C under atmosphere yields DMPP with a total yield of 65–70%.
Table 1: Optimization Parameters for Kabachnik-Fields Reaction
Parameter | Optimal Value | Effect on Yield |
---|---|---|
Reaction Temperature | 110°C | Maximizes imine formation |
Molar Ratio (P:Al:Am) | 1:1.5:0.5 | Prevents oligomerization |
Acid Catalyst | Acetic Acid (1.2 eq) | Enhances protonation |
Property | Value | Source |
---|---|---|
Melting Point | 32.5–37.5°C | |
Boiling Point | 151°C (0.8 Torr) | |
Density (25°C) | 1.21 g/mL | |
LogP | 2.8 |
Mechanistic Insights and Byproduct Analysis
Side Reactions in Pudovik Approach
Competing aldol condensation of formaldehyde can occur at temperatures >100°C, leading to polymeric byproducts. Gas chromatography-mass spectrometry (GC-MS) analysis reveals trace amounts of diphenyl phosphate (<3%) due to phosphite oxidation .
Hydrolysis Stability
DMPP exhibits moderate hydrolysis resistance (1.56 g/L solubility in water at 20°C) , but prolonged exposure to alkaline conditions cleaves the P–O–Ph bonds, regenerating methylphosphonic acid.
Industrial-Scale Production Considerations
Catalytic Efficiency
Magnesia in the Pudovik reaction offers a recyclable catalyst, achieving a turnover number (TON) of 12.3 after five cycles . In contrast, pyridine in esterification necessitates neutralization, increasing waste generation.
Environmental Impact
The Kabachnik-Fields route generates stoichiometric HBr, requiring neutralization (e.g., with ) to minimize ecological footprint .
Analyse Des Réactions Chimiques
Types of Reactions
Diphenyl methylphosphonate undergoes various chemical reactions, including:
Oxidation: It can be oxidized to form diphenyl phosphonate.
Reduction: Reduction reactions can convert it to diphenyl methylphosphine.
Substitution: It can undergo nucleophilic substitution reactions where the phenyl groups are replaced by other nucleophiles.
Common Reagents and Conditions
Oxidation: Common oxidizing agents include hydrogen peroxide and potassium permanganate.
Reduction: Reducing agents such as lithium aluminum hydride are used.
Substitution: Nucleophiles like alkoxides and amines are commonly used in substitution reactions.
Major Products Formed
Oxidation: Diphenyl phosphonate.
Reduction: Diphenyl methylphosphine.
Substitution: Various substituted phosphonates depending on the nucleophile used.
Applications De Recherche Scientifique
Applications Overview
-
Flame Retardant Additive
- DPMP is widely used as a flame retardant in polymer formulations. It enhances the fire resistance of materials, particularly polyurethane foams.
- Case Study : Research indicates that incorporating DPMP into crosslinked polyurethane increases mechanical properties and flame retardancy, making it suitable for applications in construction and automotive industries .
-
Chemical Synthesis
- DPMP serves as a reagent in organic synthesis, particularly for the preparation of phosphonates and phosphonic acids.
- Mechanistic Studies : Studies have shown that DPMP can facilitate bond cleavages in various synthetic pathways, providing a means to generate valuable intermediates in pharmaceutical chemistry .
-
Hydrolysis Studies
- The hydrolysis of DPMP has been extensively studied to understand its degradation pathways and reactivity under different conditions. This research is crucial for assessing environmental impacts and developing safer chemical processes.
- Findings : Hydrolysis rates were found to vary significantly with pH and temperature, influencing the stability of DPMP in various applications .
Data Tables
Case Studies
- Flame Retardant Efficacy :
-
Synthetic Pathways :
- Research exploring the catalytic properties of DPMP in bond cleavages has revealed its potential in synthesizing complex organic molecules. Control experiments indicated that varying reaction conditions significantly affects yield and selectivity, highlighting its utility in fine chemical synthesis .
Mécanisme D'action
The mechanism of action of diphenyl methylphosphonate involves its interaction with molecular targets through its phosphorus atom. It can form strong bonds with oxygen and nitrogen atoms in biological molecules, affecting their structure and function. The compound can also undergo hydrolysis to release phosphonic acid derivatives, which can interact with enzymes and other proteins .
Comparaison Avec Des Composés Similaires
Substituted Diphenyl Aminophosphonates
Structural modifications significantly influence biological activity:
- Pyrene-derived analogs : The presence of pyrene enhances cytotoxicity against cancer cell lines but increases ecotoxicity. For example, 3d (with a benzyl group) is more toxic to H. incongruens (80% mortality at 50 mg/kg) than 3b (with a methoxyphenyl group) .
- Thiophene-derived analogs : Substitution with thiophene moieties reduces herbicidal potency. Compound 1 inhibits weed growth by ≤59.4% but harms radish (EC₅₀ = 523.1–956 mg/kg) and oat roots (EC₅₀ = 611.5 mg/kg) .
Alkyl Methylphosphonates
Compounds like dimethyl methylphosphonate (DMMP) and diethyl methylphosphonate are structurally simpler simulants for organophosphorus nerve agents. They are chosen for their low toxicity and physicochemical similarities to hazardous agents . DMMP, for instance, is non-interfering in molecularly imprinted polymer studies even at 1000× concentrations of target analytes .
Organophosphate Simulants
Trimethyl phosphate and triethyl phosphate are common simulants due to their volatility and hydrolytic stability. Unlike this compound derivatives, they lack aromatic substituents, resulting in lower bioactivity and environmental persistence .
Structural and Functional Insights
- Aromatic vs. Alkyl Groups : Diphenyl derivatives exhibit higher cytotoxicity and ecotoxicity than alkyl analogs due to aromatic stacking and increased hydrophobicity .
- Substituent Effects : Electron-donating groups (e.g., methoxy in 3b ) reduce toxicity compared to electron-withdrawing or bulky groups (e.g., benzyl in 3d ) .
- Applications : While alkyl methylphosphonates serve as simulants , diphenyl derivatives are explored for anticancer and agrochemical applications .
Activité Biologique
Diphenyl methylphosphonate (DPM) is a compound with significant biological activity, particularly in the fields of agrochemistry and materials science. This article provides an overview of its biological properties, including its effects on plant growth, potential as a pesticide, and implications in flame retardancy.
This compound is classified as an organophosphorus compound with the chemical formula and a CAS number of 7526-26-3. It is primarily used as an intermediate in the synthesis of various organophosphorus compounds, including potential pesticides and flame retardants. Its structure allows it to interact with biological systems effectively.
1. Phytotoxicity
Research has demonstrated that this compound exhibits phytotoxic effects on various plant species. A study assessed its impact on seedling emergence and growth in monocotyledonous and dicotyledonous plants, revealing significant inhibition ratios (IR) in treated plants compared to controls. The phytotoxicity was evaluated using standard OECD guidelines, indicating that DPM can affect photosynthetic pigment content and overall plant health.
Plant Species | Inhibition Ratio (%) | Comments |
---|---|---|
Raphanus sativus | 35% | Moderate phytotoxicity |
Avena sativa | 50% | High sensitivity observed |
Galinsoga parviflora | 60% | Strong inhibition noted |
2. Pesticidal Potential
This compound has been investigated for its potential as a pesticide. The compound has shown moderate insecticidal activity against various pests when tested in vitro. For instance, bioassays indicated that certain derivatives of DPM exhibited effective fungicidal properties against plant pathogens such as Physalospora piricola and Sclerotinia sclerotiorum, with effective concentrations (EC50) comparable to commercial fungicides.
Pathogen | EC50 (μg/mL) | Activity Level |
---|---|---|
Physalospora piricola | 18.8 | Comparable to triadimefon (24.7) |
Sclerotinia sclerotiorum | 30.1 | Moderate efficacy observed |
3. Toxicological Profile
The toxicity profile of this compound indicates potential risks associated with its use. It has been classified as a poison by various regulatory agencies due to its acute toxicity in certain biological assays. The compound's persistence in the environment raises concerns about bioaccumulation and long-term ecological effects.
Case Studies
Several case studies have highlighted the implications of this compound in agricultural practices:
- Case Study 1: A field trial assessing the efficacy of DPM-based formulations revealed a significant reduction in pest populations while also noting adverse effects on non-target plant species.
- Case Study 2: Laboratory studies demonstrated that DPM can enhance flame retardancy in polyurethane materials, suggesting its dual role as both an agrochemical and a material additive.
Q & A
Basic Research Questions
Q. What are the common synthetic routes and characterization techniques for diphenyl methylphosphonate (DMP) and its derivatives?
DMP is synthesized via nucleophilic substitution or condensation reactions. For example, derivatives with pyridinyl and aryl amino groups are prepared by reacting substituted anilines with pyridine-3-carboxaldehyde and methylphosphonic acid under reflux conditions, followed by purification via recrystallization . Key characterization methods include:
- Spectroscopy : and NMR to confirm molecular structure and phosphorus environment (e.g., δ 15–16 ppm for P=O groups) .
- Mass spectrometry : EI–MS or AP+–MS to verify molecular ion peaks (e.g., m/z 432–453 for substituted DMP derivatives) .
- Elemental analysis : Validation of C, H, N, and P content (e.g., ±0.3% deviation from theoretical values) .
Q. What are the key physicochemical properties of DMP relevant to experimental design?
DMP (CAS 7526-26-3) has a molecular weight of 248.21 g/mol, melting point of 32.5–37.5°C, and density of 1.21 g/mL at 25°C. It is sparingly soluble in water (1.56 g/L at 20°C) but miscible in organic solvents like chloroform and ethyl acetate. Its logP value of 2.8 indicates moderate hydrophobicity, influencing solvent selection for reactions .
Q. How is DMP utilized in the development of antimicrobial agents?
DMP derivatives (e.g., diphenyl (aryl amino)(pyridin-3-yl)methylphosphonates) exhibit antimicrobial activity by disrupting bacterial cell membranes. Structure-activity relationship (SAR) studies show that electron-withdrawing substituents (e.g., Cl, OCH) enhance potency. For example, compound 3 (4-chloro derivative) shows 100% efficacy against S. aureus at 50 µg/mL, validated via MIC assays and time-kill studies .
Advanced Research Questions
Q. What mechanistic insights explain the hydrolysis pathways of DMP and its derivatives?
Hydrolysis of DMP proceeds via an associative mechanism with a pentacoordinate phosphorane intermediate. Kinetic isotope effect (KIE) studies (e.g., ) and linear free-energy relationships (LFERs) indicate simultaneous bond formation (P–O) and fission (P–OAr). Substitutents like methyl groups stabilize the transition state, reducing hydrolysis rates by 8% compared to unsubstituted analogs .
Q. How do microbial systems contribute to the environmental occurrence of methylphosphonates like DMP?
Marine archaea and bacteria produce methylphosphonate as a phosphorus source under phosphate-limited conditions. Isotopic labeling studies show microbial degradation releases methane, linking DMP to oceanic methane paradoxes. For example, Pelagibacter ubique metabolizes DMP via C–P lyase pathways, producing CH and phosphite .
Q. What advanced analytical methods enable trace detection of DMP in complex matrices?
Molecularly imprinted polymers (MIPs) functionalized with DMP-specific binding sites achieve detection limits of 0.1 ppb in environmental samples. Interference studies using diazinon and dimethyl methylphosphonate (100–1000× excess) confirm selectivity, validated via HPLC-MS/MS with collision-induced dissociation (CID) .
Q. How can researchers resolve contradictions in kinetic data for DMP hydrolysis across studies?
Discrepancies arise from solvent effects (e.g., acidic vs. neutral conditions) and coordination with metal ions (e.g., Co(III)). To address this:
- Perform -labeling experiments to track solvent incorporation into intermediates .
- Compare LFERs for DMP and isoelectronic phosphate esters to distinguish associative/dissociative pathways .
Q. What role does DMP play in coordination chemistry and catalysis?
DMP acts as a bidentate ligand in Co(III) complexes, stabilizing transition states during ester hydrolysis. X-ray crystallography reveals distorted trigonal bipyramidal geometries in phosphorane intermediates, with bond angles (O–P–O = 90–120°) influencing catalytic efficiency .
Q. How do environmental factors influence the stability and degradation products of DMP?
Photolysis and microbial degradation are primary degradation pathways. Under UV light (λ = 254 nm), DMP decomposes into phenyl phosphate and methane. In soil, Pseudomonas spp. metabolize DMP into non-toxic phosphonate esters, monitored via NMR and GC-MS .
Q. Methodological Recommendations
- Synthesis Optimization : Use anhydrous conditions and TLC monitoring to improve yields (e.g., 81–89% for aryl amino derivatives) .
- Data Validation : Cross-reference NMR shifts with computational models (e.g., DFT for chemical shifts) .
- Environmental Sampling : Employ solid-phase extraction (SPE) with C18 cartridges to isolate DMP from aqueous matrices .
Propriétés
IUPAC Name |
[methyl(phenoxy)phosphoryl]oxybenzene | |
---|---|---|
Source | PubChem | |
URL | https://pubchem.ncbi.nlm.nih.gov | |
Description | Data deposited in or computed by PubChem | |
InChI |
InChI=1S/C13H13O3P/c1-17(14,15-12-8-4-2-5-9-12)16-13-10-6-3-7-11-13/h2-11H,1H3 | |
Source | PubChem | |
URL | https://pubchem.ncbi.nlm.nih.gov | |
Description | Data deposited in or computed by PubChem | |
InChI Key |
HPUPGAFDTWIMBR-UHFFFAOYSA-N | |
Source | PubChem | |
URL | https://pubchem.ncbi.nlm.nih.gov | |
Description | Data deposited in or computed by PubChem | |
Canonical SMILES |
CP(=O)(OC1=CC=CC=C1)OC2=CC=CC=C2 | |
Source | PubChem | |
URL | https://pubchem.ncbi.nlm.nih.gov | |
Description | Data deposited in or computed by PubChem | |
Molecular Formula |
C13H13O3P | |
Source | PubChem | |
URL | https://pubchem.ncbi.nlm.nih.gov | |
Description | Data deposited in or computed by PubChem | |
DSSTOX Substance ID |
DTXSID2064731 | |
Record name | Diphenyl methylphosphonate | |
Source | EPA DSSTox | |
URL | https://comptox.epa.gov/dashboard/DTXSID2064731 | |
Description | DSSTox provides a high quality public chemistry resource for supporting improved predictive toxicology. | |
Molecular Weight |
248.21 g/mol | |
Source | PubChem | |
URL | https://pubchem.ncbi.nlm.nih.gov | |
Description | Data deposited in or computed by PubChem | |
CAS No. |
7526-26-3 | |
Record name | Diphenyl methylphosphonate | |
Source | CAS Common Chemistry | |
URL | https://commonchemistry.cas.org/detail?cas_rn=7526-26-3 | |
Description | CAS Common Chemistry is an open community resource for accessing chemical information. Nearly 500,000 chemical substances from CAS REGISTRY cover areas of community interest, including common and frequently regulated chemicals, and those relevant to high school and undergraduate chemistry classes. This chemical information, curated by our expert scientists, is provided in alignment with our mission as a division of the American Chemical Society. | |
Explanation | The data from CAS Common Chemistry is provided under a CC-BY-NC 4.0 license, unless otherwise stated. | |
Record name | Phosphonic acid, P-methyl-, diphenyl ester | |
Source | ChemIDplus | |
URL | https://pubchem.ncbi.nlm.nih.gov/substance/?source=chemidplus&sourceid=0007526263 | |
Description | ChemIDplus is a free, web search system that provides access to the structure and nomenclature authority files used for the identification of chemical substances cited in National Library of Medicine (NLM) databases, including the TOXNET system. | |
Record name | Phosphonic acid, P-methyl-, diphenyl ester | |
Source | EPA Chemicals under the TSCA | |
URL | https://www.epa.gov/chemicals-under-tsca | |
Description | EPA Chemicals under the Toxic Substances Control Act (TSCA) collection contains information on chemicals and their regulations under TSCA, including non-confidential content from the TSCA Chemical Substance Inventory and Chemical Data Reporting. | |
Record name | Diphenyl methylphosphonate | |
Source | EPA DSSTox | |
URL | https://comptox.epa.gov/dashboard/DTXSID2064731 | |
Description | DSSTox provides a high quality public chemistry resource for supporting improved predictive toxicology. | |
Record name | Diphenyl methylphosphonate | |
Source | European Chemicals Agency (ECHA) | |
URL | https://echa.europa.eu/substance-information/-/substanceinfo/100.028.535 | |
Description | The European Chemicals Agency (ECHA) is an agency of the European Union which is the driving force among regulatory authorities in implementing the EU's groundbreaking chemicals legislation for the benefit of human health and the environment as well as for innovation and competitiveness. | |
Explanation | Use of the information, documents and data from the ECHA website is subject to the terms and conditions of this Legal Notice, and subject to other binding limitations provided for under applicable law, the information, documents and data made available on the ECHA website may be reproduced, distributed and/or used, totally or in part, for non-commercial purposes provided that ECHA is acknowledged as the source: "Source: European Chemicals Agency, http://echa.europa.eu/". Such acknowledgement must be included in each copy of the material. ECHA permits and encourages organisations and individuals to create links to the ECHA website under the following cumulative conditions: Links can only be made to webpages that provide a link to the Legal Notice page. | |
Synthesis routes and methods I
Procedure details
Synthesis routes and methods II
Procedure details
Retrosynthesis Analysis
AI-Powered Synthesis Planning: Our tool employs the Template_relevance Pistachio, Template_relevance Bkms_metabolic, Template_relevance Pistachio_ringbreaker, Template_relevance Reaxys, Template_relevance Reaxys_biocatalysis model, leveraging a vast database of chemical reactions to predict feasible synthetic routes.
One-Step Synthesis Focus: Specifically designed for one-step synthesis, it provides concise and direct routes for your target compounds, streamlining the synthesis process.
Accurate Predictions: Utilizing the extensive PISTACHIO, BKMS_METABOLIC, PISTACHIO_RINGBREAKER, REAXYS, REAXYS_BIOCATALYSIS database, our tool offers high-accuracy predictions, reflecting the latest in chemical research and data.
Strategy Settings
Precursor scoring | Relevance Heuristic |
---|---|
Min. plausibility | 0.01 |
Model | Template_relevance |
Template Set | Pistachio/Bkms_metabolic/Pistachio_ringbreaker/Reaxys/Reaxys_biocatalysis |
Top-N result to add to graph | 6 |
Feasible Synthetic Routes
Avertissement et informations sur les produits de recherche in vitro
Veuillez noter que tous les articles et informations sur les produits présentés sur BenchChem sont destinés uniquement à des fins informatives. Les produits disponibles à l'achat sur BenchChem sont spécifiquement conçus pour des études in vitro, qui sont réalisées en dehors des organismes vivants. Les études in vitro, dérivées du terme latin "in verre", impliquent des expériences réalisées dans des environnements de laboratoire contrôlés à l'aide de cellules ou de tissus. Il est important de noter que ces produits ne sont pas classés comme médicaments et n'ont pas reçu l'approbation de la FDA pour la prévention, le traitement ou la guérison de toute condition médicale, affection ou maladie. Nous devons souligner que toute forme d'introduction corporelle de ces produits chez les humains ou les animaux est strictement interdite par la loi. Il est essentiel de respecter ces directives pour assurer la conformité aux normes légales et éthiques en matière de recherche et d'expérimentation.