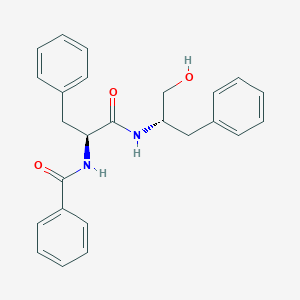
Aurantiamide
Vue d'ensemble
Description
Aurantiamide is an orally active constituent of Portulaca oleracea L and has various biological activities, including antioxidant, antiplatelet, anti-inflammatory, and antitumor activities .
Synthesis Analysis
Aurantiamide and aurantiamide acetate are new amides from Piper aurantiacum Wall . A series of new aurantiamide acetate analogues were synthesized by modifying its N-terminal substitution and the amino acid residue .
Molecular Structure Analysis
Aurantiamide has a molecular formula of C25H26N2O3 . It is a natural product found in Piper wallichii, Portulaca oleracea, and other organisms . Aurantiamide acetate is a dipeptide derivative with a structure that resembles an endogenous peptide found in both tissues and plasma .
Chemical Reactions Analysis
Aurantiamide and aurantiamide acetate were rapidly absorbed following oral administration, both achieving a Cmax at around 0.2 h . The extent of their metabolisms also varied among different organ tissues, resulting in about 90% reduction in concentrations 4 h after their administration .
Physical And Chemical Properties Analysis
Aurantiamide has a molecular weight of 402.5 g/mol . The concentrations of aurantiamide and aurantiamide acetate in the plasma and various organ tissues of rat were quantitated using a validated UHPLC-MS/MS method .
Applications De Recherche Scientifique
Inhibition of Cysteine Proteinases
Aurantiamide acetate has been identified as a selective inhibitor of cysteine proteinases, particularly cathepsin L and B . This inhibition is significant in the study of diseases like osteoarthritis and rheumatoid arthritis, where cysteine proteinases play a role in tissue degradation.
Analgesic and Anti-inflammatory Agents
Research has shown that aurantiamide acetate analogues can be synthesized to create potent analgesic and anti-inflammatory agents . These compounds have been evaluated for their effectiveness in reducing pain and inflammation in animal models, showing promise for future therapeutic applications.
Suppression of Malignant Gliomas
Aurantiamide acetate has demonstrated the ability to suppress the growth of malignant gliomas both in vitro and in vivo by inhibiting autophagic flux . This suggests a potential role for aurantiamide in developing treatments for aggressive brain cancers.
Neuroinflammation Modulation
Aurantiamide has been studied for its effects on neuroinflammation, showing potential to improve cognitive functions by targeting the NLRP3 inflammasome, which is implicated in microglial cell polarization and inflammation in the brain .
Lung Inflammation and Acute Lung Injury
In the context of lung health, aurantiamide acetate has been found to ameliorate lung inflammation in models of acute lung injury, suggesting a protective effect that could be harnessed for treating conditions like ALI and possibly other respiratory ailments .
Antiviral Effects
Aurantiamide acetate has exhibited antiviral effects by inhibiting the NF-κB signaling pathway in cells infected with the Influenza A virus . This highlights its potential use in the development of new antiviral therapies.
Mécanisme D'action
Target of Action
Aurantiamide primarily targets cathepsin K (CTSK) and matrix metallopeptidase 9 (MMP9) . These proteins play a significant role in the regulation of osteoporosis . Aurantiamide also interacts with formyl peptide receptor 1 (FPR1) , a key regulator of the inflammatory environment .
Mode of Action
Aurantiamide interacts with its targets, leading to various changes. It inhibits cysteine proteinases, particularly cathepsin L and B . It also acts as an antagonist for FPR1, modulating innate immunity and potentially treating inflammatory diseases .
Biochemical Pathways
Aurantiamide affects several biochemical pathways. It suppresses the activation of NF-κB and PI3K/AKT phosphorylation , which are involved in inflammation and cell survival. By inhibiting CTSK and MMP9, aurantiamide impacts biological processes and signaling pathways associated with the regulation of osteoporosis .
Pharmacokinetics
Aurantiamide and its derivative, aurantiamide acetate, are rapidly absorbed following oral administration, achieving peak concentration around 0.2 hours . Their metabolism varies among different organ tissues, resulting in about a 90% reduction in concentrations 4 hours after administration . This indicates that there is no long-term accumulation in the tissues .
Result of Action
Aurantiamide has several molecular and cellular effects. It significantly reduces lung pathological changes, water-to-dry (W/D) ratio, myeloperoxidase (MPO) activity, and protein content . It also results in a significant reduction in the number of total cells, neutrophils, and proinflammatory cytokines in the bronchoalveolar lavage fluid (BALF) after lipopolysaccharide (LPS) stimulation .
Action Environment
The action of aurantiamide can be influenced by environmental factors. For instance, the inflammatory environment can affect the interaction of aurantiamide with FPR1
Orientations Futures
Propriétés
IUPAC Name |
N-[(2S)-1-[[(2S)-1-hydroxy-3-phenylpropan-2-yl]amino]-1-oxo-3-phenylpropan-2-yl]benzamide | |
---|---|---|
Source | PubChem | |
URL | https://pubchem.ncbi.nlm.nih.gov | |
Description | Data deposited in or computed by PubChem | |
InChI |
InChI=1S/C25H26N2O3/c28-18-22(16-19-10-4-1-5-11-19)26-25(30)23(17-20-12-6-2-7-13-20)27-24(29)21-14-8-3-9-15-21/h1-15,22-23,28H,16-18H2,(H,26,30)(H,27,29)/t22-,23-/m0/s1 | |
Source | PubChem | |
URL | https://pubchem.ncbi.nlm.nih.gov | |
Description | Data deposited in or computed by PubChem | |
InChI Key |
KSVKECXWDNCRTM-GOTSBHOMSA-N | |
Source | PubChem | |
URL | https://pubchem.ncbi.nlm.nih.gov | |
Description | Data deposited in or computed by PubChem | |
Canonical SMILES |
C1=CC=C(C=C1)CC(CO)NC(=O)C(CC2=CC=CC=C2)NC(=O)C3=CC=CC=C3 | |
Source | PubChem | |
URL | https://pubchem.ncbi.nlm.nih.gov | |
Description | Data deposited in or computed by PubChem | |
Isomeric SMILES |
C1=CC=C(C=C1)C[C@@H](CO)NC(=O)[C@H](CC2=CC=CC=C2)NC(=O)C3=CC=CC=C3 | |
Source | PubChem | |
URL | https://pubchem.ncbi.nlm.nih.gov | |
Description | Data deposited in or computed by PubChem | |
Molecular Formula |
C25H26N2O3 | |
Source | PubChem | |
URL | https://pubchem.ncbi.nlm.nih.gov | |
Description | Data deposited in or computed by PubChem | |
DSSTOX Substance ID |
DTXSID90893261 | |
Record name | Aurantiamide | |
Source | EPA DSSTox | |
URL | https://comptox.epa.gov/dashboard/DTXSID90893261 | |
Description | DSSTox provides a high quality public chemistry resource for supporting improved predictive toxicology. | |
Molecular Weight |
402.5 g/mol | |
Source | PubChem | |
URL | https://pubchem.ncbi.nlm.nih.gov | |
Description | Data deposited in or computed by PubChem | |
Product Name |
Aurantiamide | |
CAS RN |
58115-31-4 | |
Record name | N-((1S)-1-(((2S)-1-Hydroxy-3-phenyl-propan-2-yl)carbamoyl)-2-phenyl-ethyl)benzamide | |
Source | ChemIDplus | |
URL | https://pubchem.ncbi.nlm.nih.gov/substance/?source=chemidplus&sourceid=0058115314 | |
Description | ChemIDplus is a free, web search system that provides access to the structure and nomenclature authority files used for the identification of chemical substances cited in National Library of Medicine (NLM) databases, including the TOXNET system. | |
Record name | Aurantiamide | |
Source | EPA DSSTox | |
URL | https://comptox.epa.gov/dashboard/DTXSID90893261 | |
Description | DSSTox provides a high quality public chemistry resource for supporting improved predictive toxicology. | |
Retrosynthesis Analysis
AI-Powered Synthesis Planning: Our tool employs the Template_relevance Pistachio, Template_relevance Bkms_metabolic, Template_relevance Pistachio_ringbreaker, Template_relevance Reaxys, Template_relevance Reaxys_biocatalysis model, leveraging a vast database of chemical reactions to predict feasible synthetic routes.
One-Step Synthesis Focus: Specifically designed for one-step synthesis, it provides concise and direct routes for your target compounds, streamlining the synthesis process.
Accurate Predictions: Utilizing the extensive PISTACHIO, BKMS_METABOLIC, PISTACHIO_RINGBREAKER, REAXYS, REAXYS_BIOCATALYSIS database, our tool offers high-accuracy predictions, reflecting the latest in chemical research and data.
Strategy Settings
Precursor scoring | Relevance Heuristic |
---|---|
Min. plausibility | 0.01 |
Model | Template_relevance |
Template Set | Pistachio/Bkms_metabolic/Pistachio_ringbreaker/Reaxys/Reaxys_biocatalysis |
Top-N result to add to graph | 6 |
Feasible Synthetic Routes
Q & A
Q1: What are the known biological activities of aurantiamide acetate?
A1: Aurantiamide acetate exhibits a range of biological activities, including:
- Anticancer activity: Studies have demonstrated its ability to inhibit the growth of malignant gliomas [], possibly by blocking autophagic flux.
- Anti-inflammatory activity: It has shown efficacy in reducing inflammation in models of lipopolysaccharide-induced acute lung injury [], potentially through modulation of the NF-κB and PI3K/AKT signaling pathways.
- Antinociceptive activity: Aurantiamide acetate has demonstrated pain-reducing effects. []
Q2: How does aurantiamide acetate exert its anticancer effects?
A2: Research suggests that aurantiamide acetate might suppress the growth of malignant gliomas by inhibiting autophagic flux []. This process involves the degradation and recycling of cellular components. By disrupting this process, aurantiamide acetate could potentially induce cell death in cancer cells.
Q3: Does aurantiamide acetate interact with specific molecular targets?
A3: Yes, aurantiamide acetate has been identified as a selective inhibitor of cathepsin L [, ], a lysosomal cysteine protease involved in various physiological and pathological processes.
Q4: What is the role of NLRP3 inflammasome in aurantiamide's activity?
A4: Research indicates that aurantiamide can suppress the activation of the NLRP3 inflammasome, a key component of the innate immune system involved in inflammation []. This suggests a potential mechanism for its anti-inflammatory effects.
Q5: How does aurantiamide affect microglial cells?
A5: Studies have shown that aurantiamide can promote the M2 polarization of microglial cells [], which are immune cells in the brain. M2 polarization is associated with anti-inflammatory effects and tissue repair, suggesting a potential role for aurantiamide in neurodegenerative diseases.
Q6: What is the molecular formula and weight of aurantiamide acetate?
A6: The molecular formula of aurantiamide acetate is C29H32N2O5, and its molecular weight is 488.58 g/mol. []
Q7: What spectroscopic techniques are used to characterize aurantiamide acetate?
A7: Various spectroscopic techniques, including 1H NMR, 13C NMR, 2D NMR, and MS, are commonly employed to elucidate the structure of aurantiamide acetate. [, , , ] These techniques provide valuable information about the compound's connectivity, stereochemistry, and molecular weight.
Q8: What is the absolute configuration of aurantiamide acetate from marine organisms?
A8: The absolute configuration of aurantiamide acetate isolated from the marine red alga Caloglossa leprieurii has been determined as 4S,7S. []
Q9: How do structural modifications of aurantiamide acetate affect its biological activity?
A9: Modifications to the N-terminal substitution and the amino acid residue of aurantiamide acetate have been explored to assess their impact on analgesic and anti-inflammatory activity []. These studies have identified pentapeptides and tricosamers as promising derivatives with enhanced potency compared to the parent compound.
Q10: Have any hybrid molecules incorporating aurantiamide acetate been developed?
A10: Yes, researchers have synthesized hybrid molecules combining aurantiamide acetate with isopropylated genipin, aiming to enhance its anti-inflammatory properties. These hybrids have shown promising results in inhibiting TNF-α secretion and reducing NO production, potentially through the modulation of NF-κB and MAPK signaling pathways [].
Q11: What is known about the pharmacokinetics of aurantiamide and aurantiamide acetate?
A11: A study in rats revealed that aurantiamide and aurantiamide acetate are rapidly absorbed after oral administration, reaching peak plasma concentrations (Cmax) within approximately 0.2 hours []. Both compounds undergo extensive metabolism, with concentrations decreasing by about 90% within 4 hours, indicating efficient elimination and minimal risk of tissue accumulation.
Q12: Have any biomarkers been identified for monitoring aurantiamide acetate's therapeutic effects?
A12: While specific biomarkers for aurantiamide acetate are yet to be established, research has highlighted potential targets. For instance, transcriptomic analysis of lung epithelial cells treated with aurantiamide acetate revealed significant changes in gene expression related to inflammation and adhesion, suggesting these pathways as potential areas for biomarker development [].
Q13: How is computational chemistry being utilized in aurantiamide research?
A13: Computational approaches such as molecular docking and molecular dynamics simulations are extensively used to study the interactions of aurantiamide and its derivatives with various target proteins. [, , , , , , ] These methods provide valuable insights into binding modes, affinities, and potential mechanisms of action.
Q14: Have any quantitative structure-activity relationship (QSAR) models been developed for aurantiamide derivatives?
A14: Yes, QSAR models have been developed to predict the biological activity of aurantiamide derivatives. [] These models correlate structural features of the molecules with their observed activity, aiding in the design of new and more potent analogs.
Q15: What is known about the toxicity and safety profile of aurantiamide acetate?
A15: While aurantiamide acetate has demonstrated promising biological activities, information regarding its toxicity and safety profile is limited. Further research, particularly in vivo studies, is crucial to assess potential adverse effects and determine safe and effective doses for therapeutic applications.
Q16: From which natural sources are aurantiamide and its acetate derivative obtained?
A16: Aurantiamide and aurantiamide acetate have been isolated from various plant sources, including:
- Clematis terniflora DC []
- Portulaca oleracea L. (purslane) []
- Hedyotis corymbosa Lamk. []
- Caloglossa leprieurii (red alga) []
- Dianthus superbus []
- Aspergillus penicilloides (fungus) []
- Gomphrena celosioides []
- Murraya exotica []
- Houttuynia cordata []
- Cichorium intybus L. []
- Cordyceps sinensis []
- Nerviliae fordii []
- Selaginella involven Spring []
- Ligularia narynensis []
- Arisaema erubescens []
- Sigmadocia cymiformis Esper (marine sponge) []
- Dregea volubilis []
- Areca catechu []
- Viola yedoensis [, ]
- Inula cappa []
- Vitex leptobotrys []
- Rhizoma Iridis Tectori []
- Angiopteris fokiensis []
- Artemisia lactiflora []
- Crateva adansonii DC []
- Gomphrena agrestis []
Avertissement et informations sur les produits de recherche in vitro
Veuillez noter que tous les articles et informations sur les produits présentés sur BenchChem sont destinés uniquement à des fins informatives. Les produits disponibles à l'achat sur BenchChem sont spécifiquement conçus pour des études in vitro, qui sont réalisées en dehors des organismes vivants. Les études in vitro, dérivées du terme latin "in verre", impliquent des expériences réalisées dans des environnements de laboratoire contrôlés à l'aide de cellules ou de tissus. Il est important de noter que ces produits ne sont pas classés comme médicaments et n'ont pas reçu l'approbation de la FDA pour la prévention, le traitement ou la guérison de toute condition médicale, affection ou maladie. Nous devons souligner que toute forme d'introduction corporelle de ces produits chez les humains ou les animaux est strictement interdite par la loi. Il est essentiel de respecter ces directives pour assurer la conformité aux normes légales et éthiques en matière de recherche et d'expérimentation.