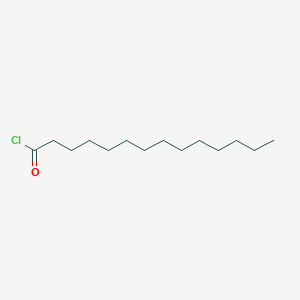
Chlorure de myristoyle
Vue d'ensemble
Description
Myristoyl chloride, also known as tetradecanoyl chloride, is an organic compound with the molecular formula CH₃(CH₂)₁₂COCl. It is a colorless to pale yellow liquid with a pungent odor. This compound is a member of the fatty acid chlorides family and is primarily used in organic synthesis, particularly in the preparation of myristoylated compounds.
Applications De Recherche Scientifique
Myristoyl chloride has a wide range of applications in scientific research:
Chemistry: It is used in the synthesis of myristoylated compounds, which are important intermediates in organic synthesis.
Biology: Myristoylated proteins play crucial roles in cellular signaling and membrane localization.
Medicine: Myristoylated compounds are investigated for their potential therapeutic applications, including drug delivery systems.
Industry: It is used in the production of surfactants, lubricants, and other industrial chemicals
Mécanisme D'action
Target of Action
Myristoyl chloride, also known as Tetradecanoyl chloride, primarily targets proteins in both eukaryotic and viral systems . The primary role of these proteins is to facilitate various biological functions, including signal transduction, cellular localization, and oncogenesis .
Mode of Action
Myristoyl chloride interacts with its protein targets through a process called N-myristoylation . This is a co-translational lipidic modification specific to the alpha-amino group of an N-terminal glycine residue of many eukaryotic and viral proteins . The process is catalyzed by the enzyme N-myristoyltransferase (NMT) . The attachment of a myristoyl group increases specific protein–protein interactions, leading to the subcellular localization of myristoylated proteins with its signaling partners .
Biochemical Pathways
The myristoylation process affects several biochemical pathways. It plays a vital role in cellular signaling pathways in several biological processes, especially in carcinogenesis and immune function . It is also involved in host defense against microbial and viral infections . Furthermore, myristoylation is a prerequisite step in initiating many immune cell signaling cascades .
Result of Action
The result of Myristoyl chloride’s action is the promotion of membrane binding . This is due to the covalent attachment of myristate, a common occurrence on a wide variety of viral and cellular proteins . This attachment plays a crucial role in promoting membrane binding, which is essential for the proteins to perform their functions .
Analyse Biochimique
Biochemical Properties
In the realm of biochemical reactions, Myristoyl chloride is vital for the process of N-myristoylation . This process is catalyzed by N-myristoyltransferases (NMTs), which are ubiquitous enzymes in eukaryotes . The attachment of a myristoyl group, facilitated by Myristoyl chloride, increases specific protein-protein interactions .
Cellular Effects
The effects of Myristoyl chloride on various types of cells and cellular processes are profound. It influences cell function by impacting cell signaling pathways, gene expression, and cellular metabolism . Specifically, protein N-myristoylation, facilitated by Myristoyl chloride, plays a crucial role in regulating innate immune responses .
Molecular Mechanism
At the molecular level, Myristoyl chloride exerts its effects through the process of N-myristoylation . This process involves the attachment of myristic acid to the N-terminal glycine of a wide range of substrate proteins . This attachment adds a certain degree of regulation to myristoylated proteins, which is named the myristoyl switch .
Temporal Effects in Laboratory Settings
It is known that the process of N-myristoylation, which involves Myristoyl chloride, generally occurs co-translationally on newly synthesized polypeptides .
Metabolic Pathways
Myristoyl chloride is involved in the metabolic pathway of protein N-myristoylation . This process is catalyzed by the enzyme N-myristoyltransferase (NMT) .
Transport and Distribution
The transport and distribution of Myristoyl chloride within cells and tissues are closely tied to the process of N-myristoylation . The attachment of a myristoyl group helps in the intracellular transport of proteins, allowing them to reach the appropriate cellular localization .
Subcellular Localization
The subcellular localization of Myristoyl chloride is linked to the proteins it helps modify. The myristoyl group, attached through the action of Myristoyl chloride, plays an important role in the localization of proteins to membranes . This includes any targeting signals or post-translational modifications that direct it to specific compartments or organelles .
Méthodes De Préparation
Synthetic Routes and Reaction Conditions: Myristoyl chloride is typically synthesized from myristic acid. The most common method involves the reaction of myristic acid with thionyl chloride in the presence of a catalyst such as N,N-dimethylformamide. The reaction is carried out under an ice bath at -5°C, followed by warming to 70°C for several hours. The product is then purified by vacuum distillation to remove any residual thionyl chloride and other impurities .
Industrial Production Methods: In industrial settings, the production of myristoyl chloride follows a similar synthetic route but on a larger scale. The process involves the use of large reactors and distillation columns to ensure high yield and purity of the final product. The reaction conditions are carefully controlled to optimize the conversion of myristic acid to myristoyl chloride.
Analyse Des Réactions Chimiques
Types of Reactions: Myristoyl chloride undergoes various chemical reactions, including:
Substitution Reactions: It reacts with nucleophiles such as amines and alcohols to form myristoylated derivatives.
Hydrolysis: In the presence of water, myristoyl chloride hydrolyzes to form myristic acid and hydrochloric acid.
Acylation: It is used in acylation reactions to introduce the myristoyl group into other compounds.
Common Reagents and Conditions:
Amines and Alcohols: These nucleophiles react with myristoyl chloride under mild conditions to form amides and esters, respectively.
Water: Hydrolysis occurs readily in the presence of water, leading to the formation of myristic acid.
Catalysts: Catalysts such as N,N-dimethylformamide are often used to enhance the reaction rate and yield.
Major Products:
Myristoylated Derivatives: These include myristoylated proteins and other organic compounds used in various applications.
Myristic Acid: Formed as a result of hydrolysis.
Comparaison Avec Des Composés Similaires
Palmitoyl Chloride: Similar to myristoyl chloride but with a longer carbon chain (C16).
Lauroyl Chloride: Has a shorter carbon chain (C12) compared to myristoyl chloride.
Stearoyl Chloride: Contains an even longer carbon chain (C18).
Uniqueness: Myristoyl chloride is unique due to its specific chain length (C14), which imparts distinct physical and chemical properties. This chain length is optimal for certain biological functions, such as protein myristoylation, making it a valuable compound in biochemical research .
Activité Biologique
Myristoyl chloride, a fatty acyl chloride derived from myristic acid, has garnered attention for its biological activity, particularly in the synthesis of derivatives that exhibit antibacterial and antifungal properties. This article delves into the biological activities associated with myristoyl chloride, highlighting its role in the development of antimicrobial agents and its mechanisms of action.
Overview of Myristoyl Chloride
Myristoyl chloride (C14H27ClO) is a saturated fatty acid derivative that serves as an important chemical intermediate. It is primarily utilized to synthesize various myristic acid derivatives, which have been shown to possess significant biological activities. The conversion of myristic acid to myristoyl chloride typically involves the reaction with thionyl chloride or oxalyl chloride, yielding a reactive acyl chloride suitable for further chemical modifications.
Antimicrobial Activity
Recent studies have demonstrated that myristoyl chloride can be employed to synthesize various myristate derivatives that exhibit notable antimicrobial properties.
Synthesis of Monosaccharide Monomyristates
One significant study involved the synthesis of monosaccharide monomyristate derivatives using myristoyl chloride. The derivatives were evaluated for their antibacterial and antifungal activities:
- Synthesis Process : Myristic acid was converted to myristoyl chloride, which was then reacted with monosaccharides (fructose, glucose, and galactose) to produce their corresponding monomyristate esters.
- Yields : The reaction yields ranged from 45.80% to 79.49% depending on the specific monosaccharide used.
- Antibacterial Activity : The synthesized esters exhibited medium antibacterial activity against gram-positive bacteria such as Staphylococcus aureus and Bacillus subtilis, while showing weaker activity against gram-negative bacteria like Escherichia coli.
- Antifungal Activity : All derivatives demonstrated significant antifungal activity against Candida albicans, with galactosyl monomyristate exhibiting the highest inhibition zones at a concentration of 2.5% .
Table 1: Antimicrobial Activity of Monosaccharide Monomyristates
Compound | Antibacterial Activity (Zone of Inhibition) | Antifungal Activity (Zone of Inhibition) |
---|---|---|
Galactosyl Monomyristate | High against S. aureus, B. subtilis | High against C. albicans |
Glucosyl Monomyristate | Moderate against E. coli | Moderate against C. albicans |
Fructosyl Monomyristate | Low | Low |
The biological activity of myristoyl derivatives can be attributed to their structural characteristics:
- Interaction with Cell Membranes : The presence of hydroxyl groups in the monosaccharide moieties facilitates interactions with bacterial cell walls through hydrogen bonding and van der Waals forces, leading to cell destabilization and lysis.
- Fungal Membrane Disruption : For antifungal activity, it is hypothesized that these compounds interact with ergosterol in fungal membranes, disrupting membrane integrity and function .
Case Studies on Myristic Acid Derivatives
Recent research has focused on the design and synthesis of novel myristic acid derivatives targeting N-myristoyltransferase (NMT), an enzyme implicated in fungal pathogenesis:
- Inhibitory Activity : Several derivatives showed promising inhibitory effects against NMT, which is essential for fungal survival. Compounds such as 3u , 3m , and 3t displayed superior antifungal capabilities against strains like Candida albicans and Aspergillus niger, outperforming standard antifungal agents like fluconazole.
- Minimal Inhibitory Concentrations (MIC) : The MIC values for these compounds were significantly lower than those for fluconazole, indicating their potential as effective antifungal agents .
Table 2: Antifungal Efficacy of Novel Myristic Acid Derivatives
Compound | Target Organism | MIC (μg/mL) | Comparison to Fluconazole |
---|---|---|---|
3u | Candida albicans | 14.38 | Better |
3m | Aspergillus niger | 14.68 | Better |
Fluconazole | - | 16.30 | - |
Propriétés
IUPAC Name |
tetradecanoyl chloride | |
---|---|---|
Source | PubChem | |
URL | https://pubchem.ncbi.nlm.nih.gov | |
Description | Data deposited in or computed by PubChem | |
InChI |
InChI=1S/C14H27ClO/c1-2-3-4-5-6-7-8-9-10-11-12-13-14(15)16/h2-13H2,1H3 | |
Source | PubChem | |
URL | https://pubchem.ncbi.nlm.nih.gov | |
Description | Data deposited in or computed by PubChem | |
InChI Key |
LPWCRLGKYWVLHQ-UHFFFAOYSA-N | |
Source | PubChem | |
URL | https://pubchem.ncbi.nlm.nih.gov | |
Description | Data deposited in or computed by PubChem | |
Canonical SMILES |
CCCCCCCCCCCCCC(=O)Cl | |
Source | PubChem | |
URL | https://pubchem.ncbi.nlm.nih.gov | |
Description | Data deposited in or computed by PubChem | |
Molecular Formula |
C14H27ClO | |
Source | PubChem | |
URL | https://pubchem.ncbi.nlm.nih.gov | |
Description | Data deposited in or computed by PubChem | |
DSSTOX Substance ID |
DTXSID3059422 | |
Record name | Tetradecanoyl chloride | |
Source | EPA DSSTox | |
URL | https://comptox.epa.gov/dashboard/DTXSID3059422 | |
Description | DSSTox provides a high quality public chemistry resource for supporting improved predictive toxicology. | |
Molecular Weight |
246.81 g/mol | |
Source | PubChem | |
URL | https://pubchem.ncbi.nlm.nih.gov | |
Description | Data deposited in or computed by PubChem | |
CAS No. |
112-64-1 | |
Record name | Tetradecanoyl chloride | |
Source | CAS Common Chemistry | |
URL | https://commonchemistry.cas.org/detail?cas_rn=112-64-1 | |
Description | CAS Common Chemistry is an open community resource for accessing chemical information. Nearly 500,000 chemical substances from CAS REGISTRY cover areas of community interest, including common and frequently regulated chemicals, and those relevant to high school and undergraduate chemistry classes. This chemical information, curated by our expert scientists, is provided in alignment with our mission as a division of the American Chemical Society. | |
Explanation | The data from CAS Common Chemistry is provided under a CC-BY-NC 4.0 license, unless otherwise stated. | |
Record name | Myristoyl chloride | |
Source | ChemIDplus | |
URL | https://pubchem.ncbi.nlm.nih.gov/substance/?source=chemidplus&sourceid=0000112641 | |
Description | ChemIDplus is a free, web search system that provides access to the structure and nomenclature authority files used for the identification of chemical substances cited in National Library of Medicine (NLM) databases, including the TOXNET system. | |
Record name | Myristoyl chloride | |
Source | DTP/NCI | |
URL | https://dtp.cancer.gov/dtpstandard/servlet/dwindex?searchtype=NSC&outputformat=html&searchlist=9417 | |
Description | The NCI Development Therapeutics Program (DTP) provides services and resources to the academic and private-sector research communities worldwide to facilitate the discovery and development of new cancer therapeutic agents. | |
Explanation | Unless otherwise indicated, all text within NCI products is free of copyright and may be reused without our permission. Credit the National Cancer Institute as the source. | |
Record name | Tetradecanoyl chloride | |
Source | EPA Chemicals under the TSCA | |
URL | https://www.epa.gov/chemicals-under-tsca | |
Description | EPA Chemicals under the Toxic Substances Control Act (TSCA) collection contains information on chemicals and their regulations under TSCA, including non-confidential content from the TSCA Chemical Substance Inventory and Chemical Data Reporting. | |
Record name | Tetradecanoyl chloride | |
Source | EPA DSSTox | |
URL | https://comptox.epa.gov/dashboard/DTXSID3059422 | |
Description | DSSTox provides a high quality public chemistry resource for supporting improved predictive toxicology. | |
Record name | Myristoyl chloride | |
Source | European Chemicals Agency (ECHA) | |
URL | https://echa.europa.eu/substance-information/-/substanceinfo/100.003.631 | |
Description | The European Chemicals Agency (ECHA) is an agency of the European Union which is the driving force among regulatory authorities in implementing the EU's groundbreaking chemicals legislation for the benefit of human health and the environment as well as for innovation and competitiveness. | |
Explanation | Use of the information, documents and data from the ECHA website is subject to the terms and conditions of this Legal Notice, and subject to other binding limitations provided for under applicable law, the information, documents and data made available on the ECHA website may be reproduced, distributed and/or used, totally or in part, for non-commercial purposes provided that ECHA is acknowledged as the source: "Source: European Chemicals Agency, http://echa.europa.eu/". Such acknowledgement must be included in each copy of the material. ECHA permits and encourages organisations and individuals to create links to the ECHA website under the following cumulative conditions: Links can only be made to webpages that provide a link to the Legal Notice page. | |
Record name | MYRISTOYL CHLORIDE | |
Source | FDA Global Substance Registration System (GSRS) | |
URL | https://gsrs.ncats.nih.gov/ginas/app/beta/substances/B9J09WN7SU | |
Description | The FDA Global Substance Registration System (GSRS) enables the efficient and accurate exchange of information on what substances are in regulated products. Instead of relying on names, which vary across regulatory domains, countries, and regions, the GSRS knowledge base makes it possible for substances to be defined by standardized, scientific descriptions. | |
Explanation | Unless otherwise noted, the contents of the FDA website (www.fda.gov), both text and graphics, are not copyrighted. They are in the public domain and may be republished, reprinted and otherwise used freely by anyone without the need to obtain permission from FDA. Credit to the U.S. Food and Drug Administration as the source is appreciated but not required. | |
Synthesis routes and methods
Procedure details
Retrosynthesis Analysis
AI-Powered Synthesis Planning: Our tool employs the Template_relevance Pistachio, Template_relevance Bkms_metabolic, Template_relevance Pistachio_ringbreaker, Template_relevance Reaxys, Template_relevance Reaxys_biocatalysis model, leveraging a vast database of chemical reactions to predict feasible synthetic routes.
One-Step Synthesis Focus: Specifically designed for one-step synthesis, it provides concise and direct routes for your target compounds, streamlining the synthesis process.
Accurate Predictions: Utilizing the extensive PISTACHIO, BKMS_METABOLIC, PISTACHIO_RINGBREAKER, REAXYS, REAXYS_BIOCATALYSIS database, our tool offers high-accuracy predictions, reflecting the latest in chemical research and data.
Strategy Settings
Precursor scoring | Relevance Heuristic |
---|---|
Min. plausibility | 0.01 |
Model | Template_relevance |
Template Set | Pistachio/Bkms_metabolic/Pistachio_ringbreaker/Reaxys/Reaxys_biocatalysis |
Top-N result to add to graph | 6 |
Feasible Synthetic Routes
Avertissement et informations sur les produits de recherche in vitro
Veuillez noter que tous les articles et informations sur les produits présentés sur BenchChem sont destinés uniquement à des fins informatives. Les produits disponibles à l'achat sur BenchChem sont spécifiquement conçus pour des études in vitro, qui sont réalisées en dehors des organismes vivants. Les études in vitro, dérivées du terme latin "in verre", impliquent des expériences réalisées dans des environnements de laboratoire contrôlés à l'aide de cellules ou de tissus. Il est important de noter que ces produits ne sont pas classés comme médicaments et n'ont pas reçu l'approbation de la FDA pour la prévention, le traitement ou la guérison de toute condition médicale, affection ou maladie. Nous devons souligner que toute forme d'introduction corporelle de ces produits chez les humains ou les animaux est strictement interdite par la loi. Il est essentiel de respecter ces directives pour assurer la conformité aux normes légales et éthiques en matière de recherche et d'expérimentation.